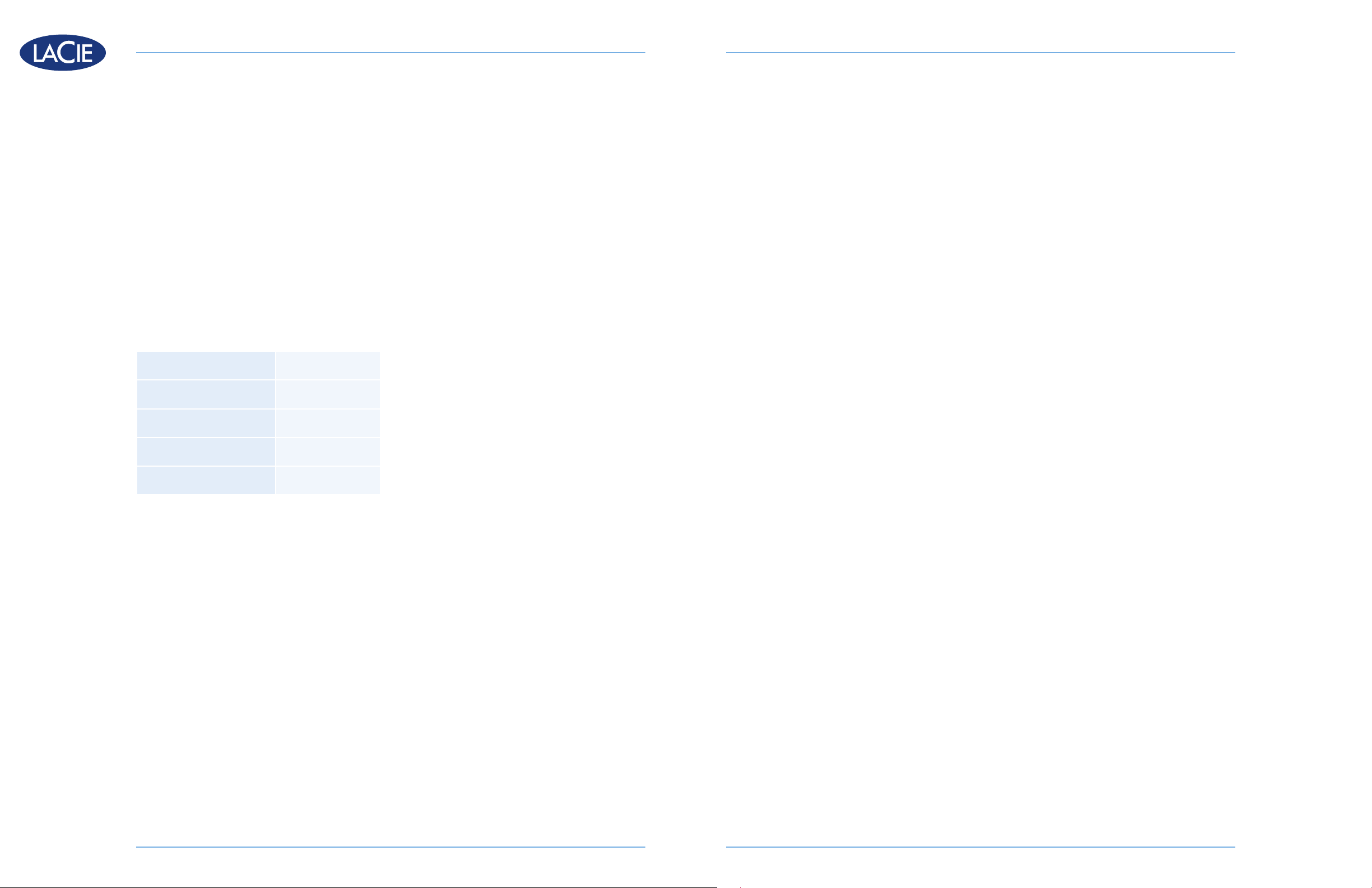
LaCie White Paper: FastKey USB 3.0 SSD
WHAT IS AN SSD?
SSD means Solid State Drive. An SSD has no moving parts and is essentially an HDD emulator based
on flash memory. It is comprised of a printed circuit
board, a set of NAND flash memory chips, SDRAM
cache, a memory controller, an interface controller,
and an interface connector such as IDE, SATA, SAS,
USB, or even fiber channel.
In addition to the performance and energy advantages of SSD, the lack of moving parts means that
the drives can withstand high vibration and shock. In
fact, some SSDs can withstand shock up to 1500G,
the equivalent of a drop from 26 feet.
Basic specifications of Solid State Drives are:
MTBF 1,000,000 hours
Data Integrity 10 years
Shock (operating) 1500G, 3 axes
Vibration (operating) 16G, each axis
Operating Temperature 0°C to 70°C
The LaCie FastKey is an SSD USB 3.0-connected
device. The FastKey uses MLC NAND flash memory
chips and two main chipsets:
✦ Indylinx barefoot: SSD controller
✦ Symwave 6316: USB 3.0 controller
MLC OR SLC, WHAT IS THE
DIFFERENCE?
Single-level cell (SLC) and multi-level cell (MLC)
Flash memory are similar in their design. MLC Flash
devices usually cost less and allow for higher storage
density. SLC Flash devices provide faster write performance and greater reliability, even at high industrial temperatures above the operating range of MLC
Flash devices. Speed performances between SLC and
MLC are comparable.
The endurance of SLC Flash is around five times that
of MLC Flash. The endurance of MLC Flash decreases during the product’s life. This is a main reason
why SLC Flash is considered industrial-grade Flash
and MLC Flash is considered consumer-grade Flash.
MLC lifetime is limited to 1,000,000 “Programmed /
Erased” cycles (10,000 cycles per cell).
HARD DRIVE DISK OR
SOLID STATE DISK?
Advantages of SSD over HDD
✦ Faster start-up because no spin-up is required.
✦ Fast random access because there is no “seek-
ing” motion as is required with rotating disk platters and the read/write head and head-actuator
mechanism
✦ Low read latency times for RAM drives. In ap-
plications where hard disk seeks are the limiting
factor, this results in faster boot and application
launch times.
✦ Consistent read performance because physical
location of data is irrelevant for SSDs.
✦ File fragmentation has negligible effect because
data access degradation due to fragmentation is
primarily due to much greater disk head seek activity, as data reads or writes are spread across many
different locations on the disk; SSDs have no heads
and thus no delays due to head motion (seeking).
✦ Silent operation due to the lack of moving parts.
✦ SSDs typically have lower power consumption
than HDDs.
✦ High mechanical reliability, as the lack of mov-
ing parts almost eliminates the risk of mechanical failure.
✦ Ability to endure extreme shock, high altitude,
vibration, and extremes of temperature.
✦ Immune to magnets.
✦ For low-capacity SSDs (like the LaCie FastKey),
lower weight and size: although size and weight
per unit storage are still better for traditional
hard drives.
✦ Failures occur less frequently while writing/eras-
ing data, which means there is a lower chance of
irrecoverable data damage.
✦ SSDs are random access by nature and can per-
form parallel reads on multiple sections of the
drive (as opposed to a HDD, which requires seek
time for each fragment, assuming a single head
assembly).
✦ Can also be configured to smaller form factors
and reduced weight.
DISADVANTAGES OF SSD
COMPARED TO HDD:
✦ Flash-memory drives have limited lifetimes and
will often wear out after 1,000,000 P/E cycles
(10,000 per cell) for MLC, and up to 5,000,000
P/E cycles (100,000 per cell) for SLC.
✦ SSDs using wear leveling cannot be defragment-
ed in order to provide maximum sequential read
speed. Optimizations do not work efficiently if
files are fragmented (access time of flash-based
SSDs is about 0.1 ms).
✦ Wear leveling used by most SSDs intrinsically in-
duces fragmentation. Moreover, defragmenting
a SSD by a defragmenter is harmful since it adds
wear to the SSD for no benefit.
✦ As of 4Q 2010, SSDs are still much more ex-
pensive per gigabyte than hard drives. Whereas
hard drives are around US$0.10 per gigabyte
for 3.5”, or US$0.20 for 2.5”, a typical flash
drive is closer to US$3 per gigabyte in 2010
✦ The maximum capacity of SSDs is currently lower
than that of hard drives.
✦ SSD write performance is significantly impacted
by the availability of free, programmable blocks.
Previously written data blocks that are no longer
in use can be reclaimed by TRIM; however, even
with TRIM, fewer free, programmable blocks
translate into reduced performance.
✦ As a result of wear leveling and write combin-
ing, the performance of SSDs degrades with use.
However, most modern SSDs now support the
TRIM command and thus return the SSD back
to its factory performance when using OSes
that support it like Windows 7, Windows Server,
2008 and Linux.
WHAT IS USB 3.0?
The Universal Serial Bus (USB) 3.0 specification is a
new industry-standard peripheral connection technology, developed by USB Implementors forum, for
connecting peripherals to PCs and laptops.
The USB 3.0 specification draws from the same architecture of the wired USB specification and therefore is a backward-compatible standard with the
same ease-of-use and plug-and-play capabilities of
previous USB technologies, but with a 10 times performance increase and lower power consumption.
The USB 3.0 specification uses two additional highspeed differential pairs for SuperSpeed mode, which
boosts its bandwidth to 5 GB/s.
For end-users of the USB 3.0 specification, the goals
of connecting peripherals with PCs or laptops are still
the same as the Hi-Speed (USB 2.0) specification, but
with significantly increased speed and reduced power
consumption.
The SuperSpeed USB specification, therefore, is not
simply an upgrade to earlier versions of the USB 2.0
specification. Due to the broad deployment of USB
2.0 devices in the market, SuperSpeed USB devices
need to be backward-compatible, but the backwardcompatibility portion of the SuperSpeed USB specification targets only the device drivers and connector
architecture. The higher speed and reduced power
consumption for the USB 3.0 specification uses advanced mechanisms and techniques similar to ones
that were used for other high bandwidth interfaces,
such as the PCI Express (PCIe) specification. As a
result, the SuperSpeed USB specification has many
differences compared to earlier generations of USB
specifications (1.1/2.0/OTG).
Page 2 Page 3
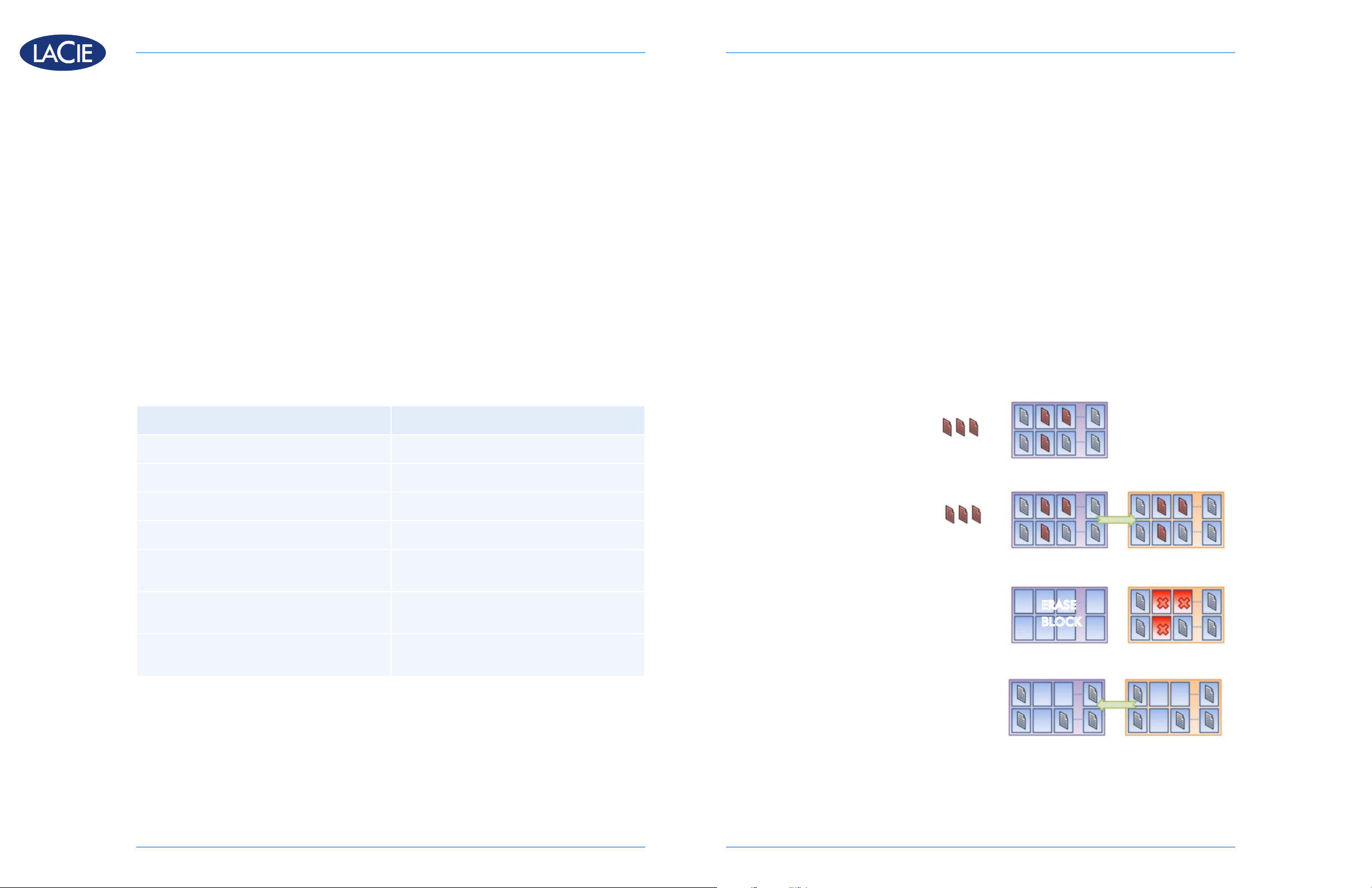
LaCie White Paper: FastKey USB 3.0 SSD
DIFFERENCES BETWEEN
SUPERSPEED USB 3.0 AND
HI-SPEED USB 2.0
The SuperSpeed USB specification is similar to earlier
USB versions in terms of the connector and device
drivers. The end-user and device driver engineer may
find SuperSpeed USB similar to earlier versions, but
it is significantly different to implementors of SuperSpeed USB host and devices.
At a mechanical level, the SuperSpeed USB specification supports dual-bus architecture for backward
compatibility to a USB 2.0 device.
This means that the SuperSpeed USB cable needs to
support eight primary wires, two wires for USB 2.0 connectors, two shared between the USB 2.0 and SuperSpeed USB specifications (PWR and GND), and four for
SuperSpeed USB dual-simplex differential signals.
SuperSpeed USB 3.0 Hi-Speed USB 2.0
Dual-simplex, unicast protocol Half-duplex, broadcast protocol
Uses asynchronous notification (NRDY, ERDY) Uses polling mechanism
Supports streaming for bulk transfers Does not support streaming
Many changes were required in the existing USB 2.0
data flow to maximize the advantages of the SuperSpeed USB bi-directional dual-simplex data interface. Though the SuperSpeed USB specification is
still a host-directed protocol and preserves the concepts of endpoints, pipes transfer types, etc., the traffic flow has changed to asynchronous as opposed to
polling traffic flow in previous USB specifications. In
addition, there are many fundamental differences at
the Protocol level as shown in the inset table.
SuperSpeed USB 3.0 also manages power consumption more efficiently, which results in some differences
at the Protocol level:
1. SuperSpeed supports link-level power management, which means either a host or a device can
initiate link power management. In USB 2.0, it is
always initiated by the host.
2. SuperSpeed USB allows isochronous devices to
enter in the low power link states between ser-
THE WEAR LEVELING
FUNCTION FOR SSD
MLC lifetime is limited to 1,000,000 “Programmed
/ Erased” cycles. LaCie Fastkey uses special static
wear levelling in order to mitigate this problem by
spreading writes over the entire device (and not always on the same memory blocks).
Static wear levelling uses a map to link the Logical
Block Addresses (LBAs) from the Operating System to
physical memory addresses. Each time the OS writes
replacement data, the map is updated so the original
physical block is marked as invalid data, and a new
block is linked to that map entry. Each time a block
of data is re-written to the Flash memory it is written
to a new location. This “rotational” effect enables the
SSD to operate until most of the blocks are near their
end of life.
TRIM FUNCTION
1
FOR SSD (TO
Pages to delete
RESIST WRITE
via TRIM
PERFORMANCE
DECLINE)
2
512KB blocks. This is important because this is the
smallest structure that can be erased. You can read
and write at a page level, but you can only erase an
entire 512KB block. This means that you can read
4KB at a time and write 4KB at a time to an empty
space, but you can’t overwrite a page. You must first
erase the content.
Windows 7 and Windows Server 2008 R2 support
the TRIM function, which they use when they detect
that a file is being deleted from an SSD. When the
OS deletes a file on an SSD, it updates the file system
but also tells the SSD via the TRIM command which
pages should be deleted. At the time of the delete,
the SSD can read the block into memory, erase the
block, and write back only pages with data in them,
as illustrated below. The delete is slower, but you
get better performance for future writes because the
pages are already empty, and write performance is
generally considered the most important factor.
NAND Block
Supports continuous bursting Does not support bursting
For OUT, token is integrated into data OUT is three separate parts (Token, Data, and
Handshake)
For IN, token is replaced by Handshake IN is three separate parts (Token, Data, and
Handshake)
Split error protection, recovery, and flow control
functionality between protocol layer and link layer
The SuperSpeed USB specification supports a dualsimplex data interface with four differential wires for
simultaneous data flow in both directions. It should
be noted that adding a bi-directional data interface
was necessary to support the SuperSpeed USB specification’s target speed because the halfduplex, two
wire differential signals of USB 2.0 and unidirectional data flow were not enough to support the SuperSpeed USB specification’s high bandwidth.
Protocol layer manages error detection, recovery,
and flow control functionality
vice intervals. This mechanism is not supported
in USB 2.0.
3. SuperSpeed USB allows devices to inform the
host of their latency tolerance using the Latency
Tolerance Messaging mechanism.
This allows the host to enter in low power states for
better power performance.
TRIM is a useful command for
Linux 2.6.33, Windows 7, and
Windows Server 2008. The LaCie FastKey supports TRIM which
improves performance when you
delete files to prepare the space
for future writing. If you overwrite
an existing file, TRIM doesn’t help
and you’ll get the same write performance as without TRIM.
SSDs behave very differently from
traditional mechanical, platterbased hard disks. SSDs are made
up of cells. These cells are organized into pages, the smallest
readable/writable unit in most
SSDs, and are normally 4KB.
These pages are then organized into blocks, traditionally 128 pages per block, for a block size of
3
4
Copy
Memory Cache
ERASE
BLOCK
Copy
Page 4 Page 5