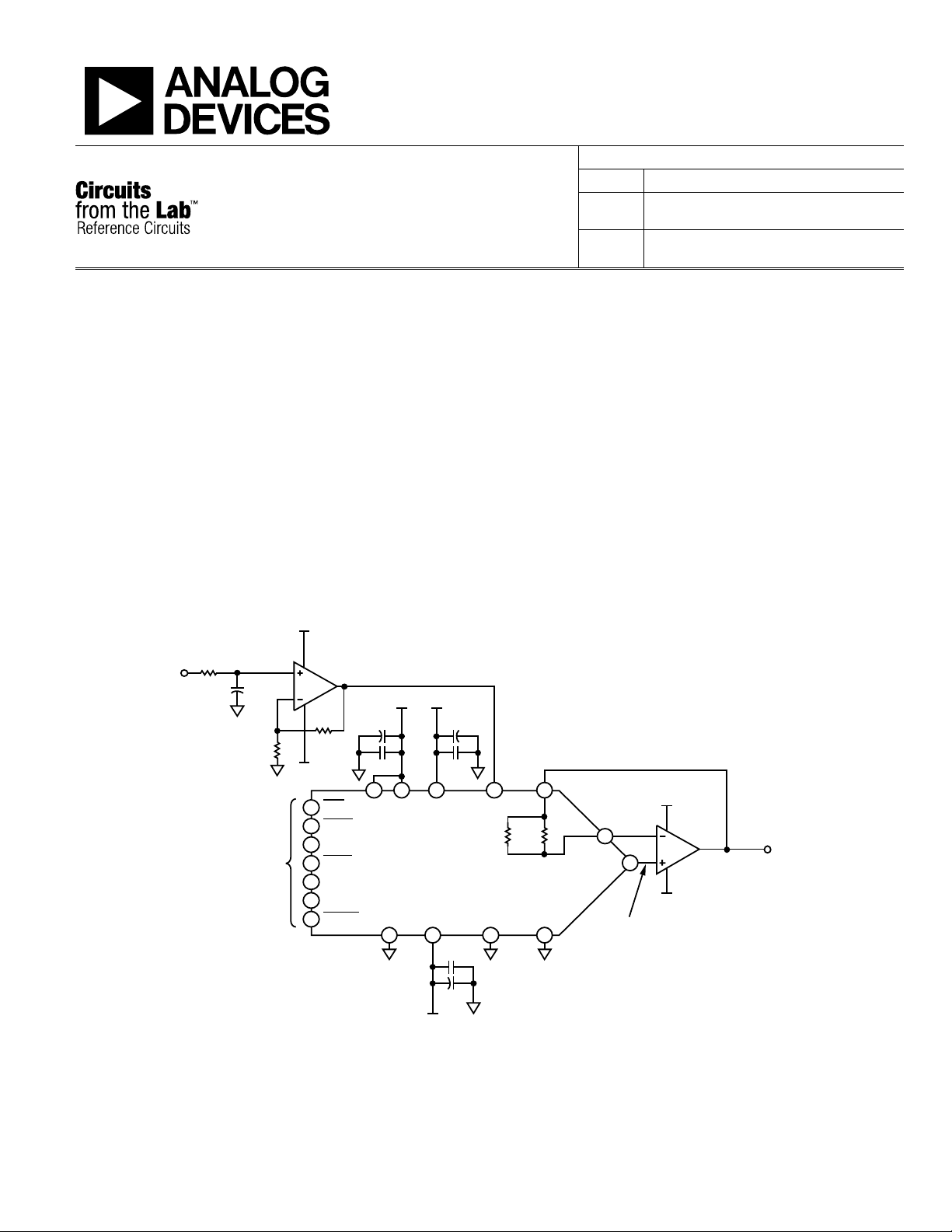
Circuit Note
Circuits from the Lab™ circuits from Analog Devices have been designed and built by Analog Devices
engineers. Standard engineering practices have been employed in the design and construction of
room temperature. However, you are solely responsible for testing the circuit and determining its
suitability and applicability for your use and application. Accordingly, in no event shall Analog Devices
iable for direct, indirect, special, incidental, consequential or punitive damages due to any cause
+
+
+
+
968 3 2
15 17 16
19
20
24
1
CLR
IOV
CCVCCVDD
V
REFP
V
SS
V
REFN
AGND
0V TO +10V
OUTPUT
VOLTAGE
0V TO +10V
DGND
V
OUT
R
FB
7
LDAC
11
SDO
14
INV
13
SCLK
SYNC
AD5790
AD8675
AD8675
12
SDIN
4
RESET
+15V
+10V
–15V
–15V
C1
10µF
A1
A2
10µF
+3.3V +15V
+15V
–15V
0.1µF
10µF
0.1µF
10µF
0.1µF
R1
1.5kΩ
TO HIGH PRECISION
+5V REFERENCE
R3
1kΩ
6.8kΩ
6.8kΩ
SPI INTERFACE
AND DIGITAL
COTNROL
R2
1kΩ
10394-001
Circuits from the Lab™ reference circuits are engineered and
tested for quick and easy system integration to help solve today’s
analog, mixed-signal, and RF design challenges. For more
information and/or support, visit www.analog.com/CN0257.
20-Bit, Linear, Low Noise, Precision, Unipolar +10 V DC Voltage Source
EVALUATION AND DESIGN SUPPORT
Circuit Evaluation Boards
AD5790 Circuit Evaluation Board (EVAL-AD5790SDZ)
System Demonstration Platform (EVAL-SDP-CB1Z)
Design and Integration Files
Schematics, Layout Files, Bill of Materials
CIRCUIT FUNCTION AND BENEFITS
The circuit, shown in Figure 1, is a 20-bit, linear, low noise,
precision, unipolar (+10 V) voltage source with a minimum
amount of external components. The AD5790 DAC is a 20-bit,
unbuffered voltage output DAC that operates from a bipolar
CN-0257
Devices Connected/Referenced
AD5790 True 20-Bit, Voltage Output DAC
AD8675
AD8676
supply of up to 33 V. The AD5790 accepts a positive reference
input range of 5 V to VDD − 2.5 V, and a negative reference input
range of VSS + 2.5 V to 0 V. Both reference inputs are buffered
on the chip, and external buffers are not required. The AD5790
offers a relative accuracy specification of ±2 LSB maximum, and
operation is guaranteed monotonic with a −1 to +2 LSB
maximum DNL specification.
The AD8675 precision op amp has low offset voltage (75 µV
maximum) and low noise (2.8 nV/√Hz typical) and is an
optimum output buffer for the AD5790. The AD5790 has two
internal matched 6.8 kΩ feedforward and feedback resistors,
which can either be connected to the AD8675 op amp to
Ultraprecision, 36 V, 2.8 nV√Hz,
Rail-to-Rail Output Op Amp
Ultraprecision, 36 V, 2.8 nV√Hz, Dual
Rail-to-Rail Output Op Amp
Rev.0
each circuit, and their function and performance have been tested and verified in a lab environment at
be l
whatsoever connected to the use of any Circuits from the Lab circuits. (Continued on last page)
Figure 1. 20-Bit Accurate, 0 V to +10 V Voltage Source (Simplified Schematic: All Connections and Decoupling Not Shown)
One Technology Way, P.O. Box 9106, Norwood, MA 02062-9106, U.S.A.
Tel: 781.329.4700
Fax: 781.461.3113 ©2011 Analog Devices, Inc. All rights reserved.
www.analog.com
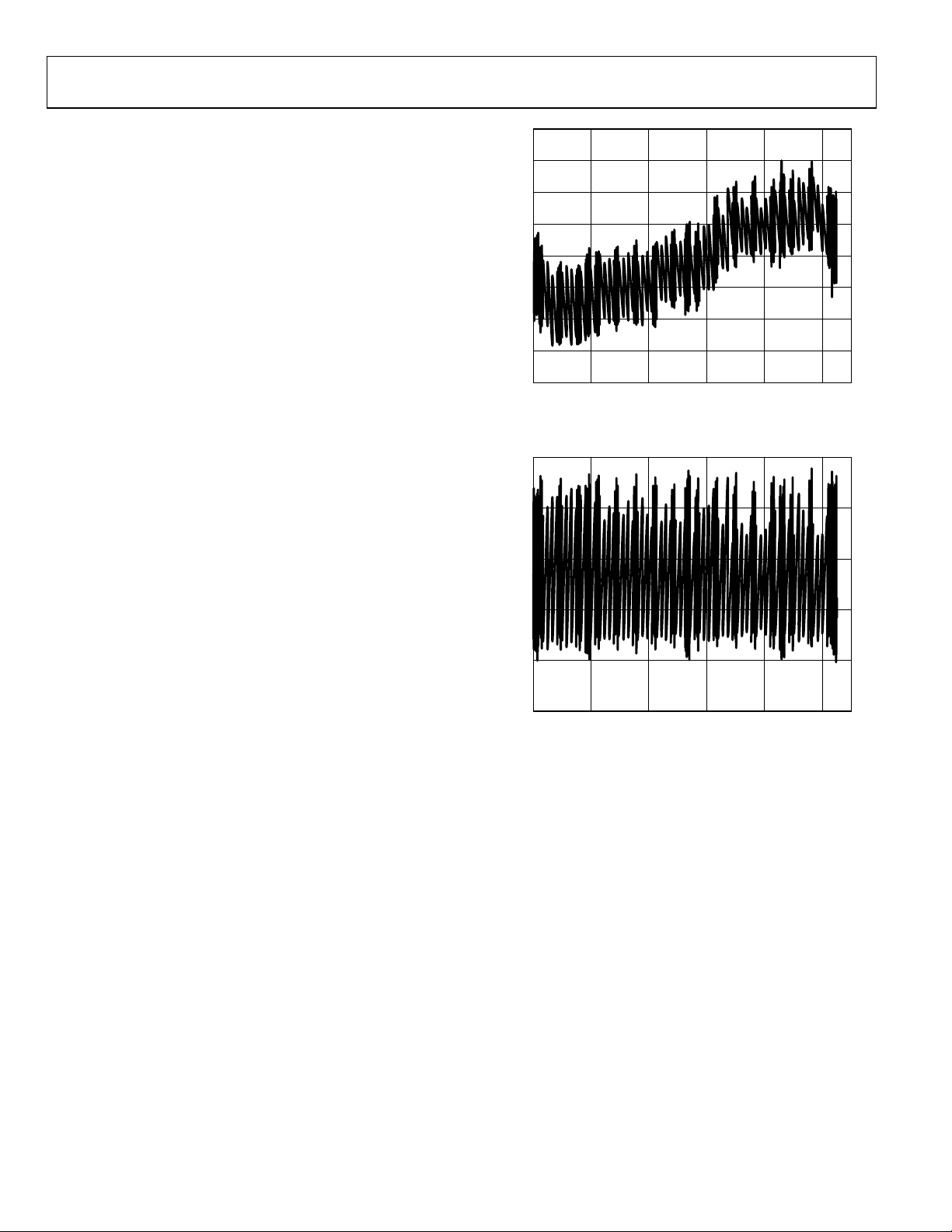
–2.0
–1.5
–1.0
–0.5
0
0.5
1.0
1.5
2.0
0 200000 400000 600000 800000 1000000
INL (LSB)
DAC CODE
10394-002
–1.0
–0.5
0
0.5
1.5
1.0
0 200000 400000 600000 800000 1000000
DNL (LSB)
DAC CODE
10394-003
CN-0257 Circuit Note
provide a 10 V offset voltage for a ±10 V output swing, or
connected in parallel to provide bias current cancellation. In
this example, a unipolar +10 V output is demonstrated, and the
resistors are used for bias current cancellation. The internal
resistor connection is controlled by setting a bit in the AD5790
control register (see AD5790 data sheet).
The digital input to the circuit is serial and is compatible with
standard SPI, QSPI, MICROWIRE®, and DSP interface standards.
For high accuracy applications, the compact circuit offers
high precision, as well as low noise—this is ensured by the
combination of the AD5790 and AD8675 precision components.
CIRCUIT DESCRIPTION
The digital-to-analog converter (DAC) shown in Figure 1 is the
AD5790, a high voltage, 20-bit converter with SPI interface,
offering ±2 LSB INL, −1 to +2 LSB DNL, and 8 nV/√Hz noise
spectral density. The AD5790 also exhibits an extremely long
term linearity error stability of 0.1 LSB.
Figure 1 shows the AD5790 in a unipolar buffered configuration.
The output buffer is the AD8675, used for its low noise and
low drift. This amplifier is also used (A1) to amplify the +5 V
reference voltage from the low noise precision reference, in this
case a Krohn Hite Model 523 precision reference. The resistors
R2 and R3 in this gain circuit are precision metal foil resistors
with 0.01% tolerance and a temperature coefficient of 0.6 ppm/°C.
For optimum performance over temperature, R2 and R3 should
be in a single package, such as the Vishay 300144 or VSR144
series. R2 and R3 are selected to be 1 kΩ to keep noise in the
system low. R1 and C1 form a low-pass filter with a cutoff
frequency of approximately 10 Hz. The purpose of this filter
is to attenuate voltage reference noise.
Linearity Measurements
The precision performance of the circuit shown in Figure 1 is
demonstrated on the EVAL -AD5790SDZ evaluation board
using an Agilent 3458A multimeter. Figure 2 shows that the
integral nonlinearity as a function of DAC code is well within
the specification of ± 2 LSB from 0°C to 105°C.
Figure 3 shows that the differential nonlinearity as a function of
DAC code is within the −1 LSB to +2 LSB specification.
Noise Drift Measurements
To be able to realize high precision, the peak-to-peak noise at
the circuit output must be maintained below 1 LSB, which is
9.5 µV for 20-bit resolution and a +10 V unipolar voltage range.
A real-time noise application will not have a high-pass cutoff at
0.1 Hz to attenuate 1/f noise but will include frequencies down
to dc in its pass band. With this in mind, the measured peak-topeak noise is shown in
output of the circuit was measured over a period of 100 seconds,
effectively including frequencies as low as 0.01 Hz in the
measurement.
A temperature controlled ultralow noise reference was required
for this measurement so as not to dominate the noise
performance.
Figure 2. Integral Nonlinearity vs. DAC Code
Figure 3. Differential Nonlinearity vs. DAC Code
Figure 4. In this case, the noise at the
Rev. 0 | Page 2 of 6