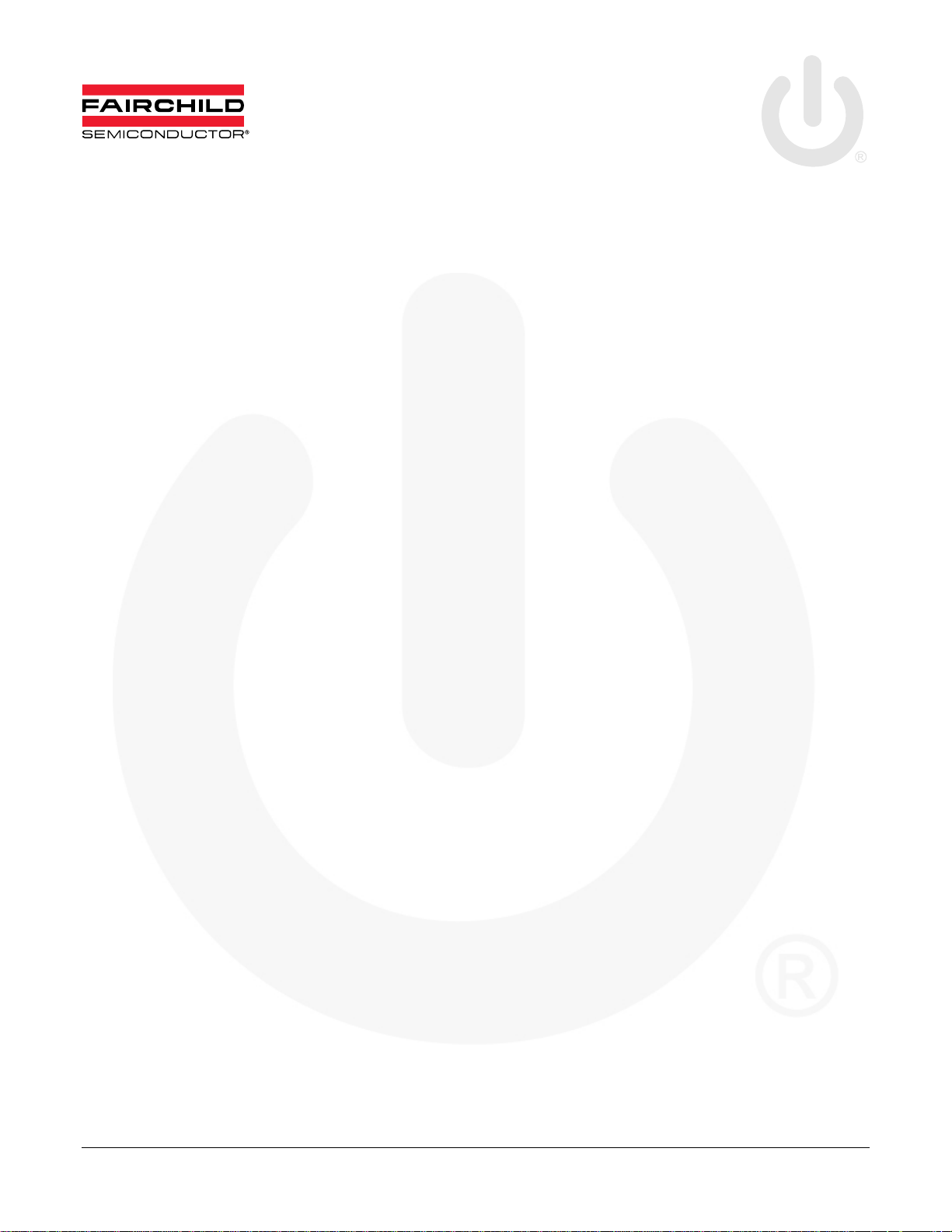
www.fairchildsemi.com
AN-6300
FAN6300 / FA N6300A / FAN6300H
Highly Integrated Quasi-Resona nt PWM Controller
Abstract
This application note describes a detailed design strategy for
higher-power conversion efficiency and better EMI using a
Quasi-Resonant PWM controller compared to the
conventional, hard-switched converter with a fixed
switching frequency. Based on the proposed design
guideline, a design example with detailed parameters
demonstrates the performance of the controller.
Introduction
The highly integrated FAN6300/A/H PWM controller
provides several features to enhance the performance of
flyback converters. FAN6300/A are applied on QuasiResonant flyback converter where maximum operating
frequency is below 100kHz and FAN6300H is suitable for
high frequency operation that is around 190kHz. A built-in
High Voltage (HV) startup circuit can provide more startup
current to reduce the startup time of the controller. Once the
VDD voltage exceeds the turn-on threshold voltage, the HV
startup function is disabled immediately to reduce power
consumption. An internal valley voltage detector ensures
power system operates in quasi-resonant operation in wide-
range line voltage and reduces switching loss to minimize
switching voltage on drain of the power MOSFET.
To minimize standby power consumption and improve lightload efficiency, a proprietary green-mode function provides
off-time modulation to decrease switching frequency and
perform extended valley voltage switching to keep to a
minimum switching voltage.
FAN6300/A/H controller provides many protection
functions. Pulse-by-pulse current limiting ensures the fixed
peak current limit level, even when short-circuit occurs.
Once an open-circuit failure occurs in the feedback loop, the
internal protection circuit disables PWM output
immediately. As long as V
threshold voltage, the controller also disables the PWM
output. The gate output is clamped at 18V to protect the
power MOS from high gate-source voltage conditions. The
minimum t
being too high. If the DET pin reaches OVP level, internal
OTP is triggered, and the power system enters latch-mode
until AC power is removed.
time limit prevents the system frequency from
OFF
drops below the turn-off
DD
© 2009 Fairchild Semiconductor Corporation www.fairchildsemi.com
Rev. 1.0.2 • 5/21/10
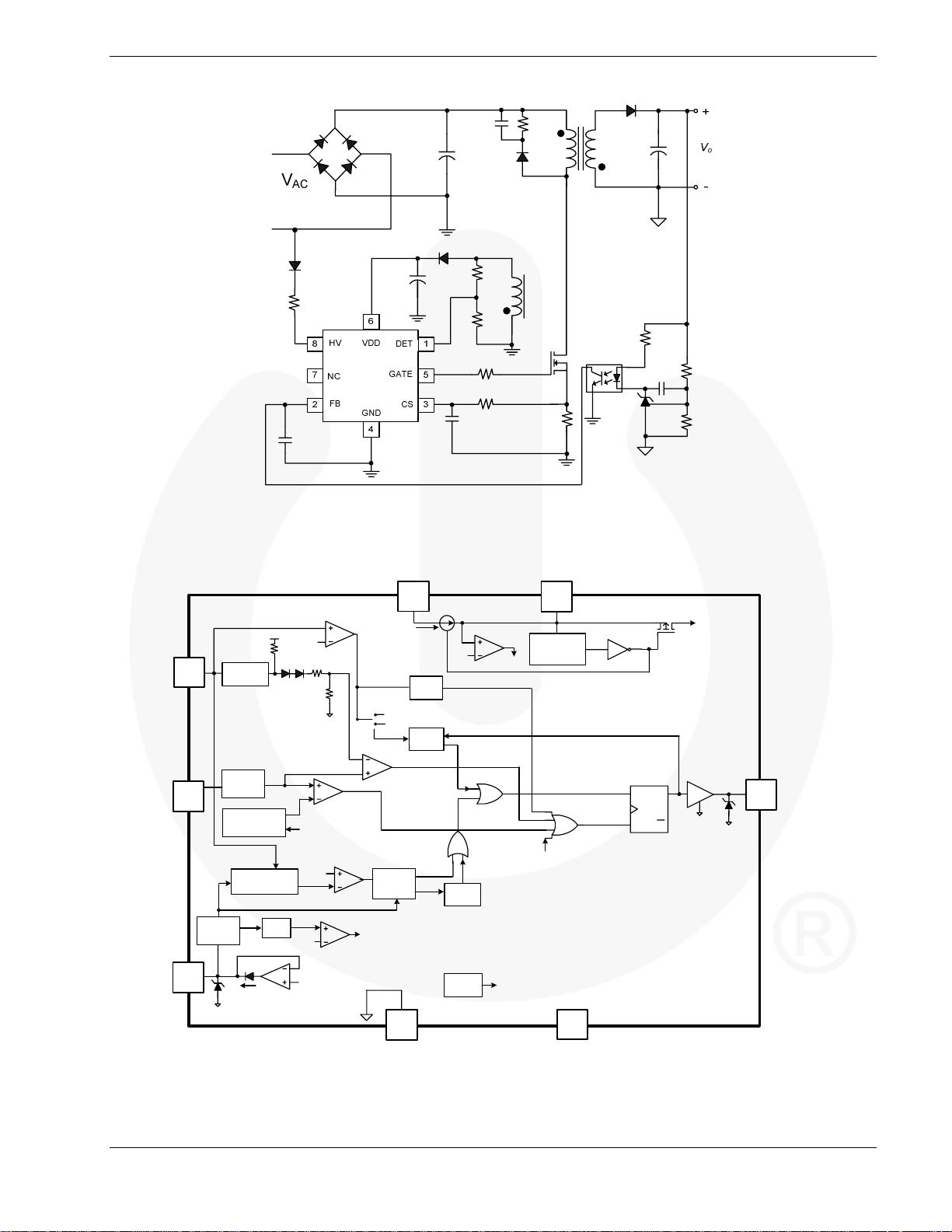
AN-6300 APPLICATION NOTE
Figure 1. Basic Quasi-Resonant Converter
FB
CS
DET
2
3
1
Soft-Start
5ms
Blanking
Circuit
Over-Power
Compensation
(3µs/13µs)
for H version
t
OFF
Blanking
(4µs)
(1.5µs) for H version
5V
t
OFF-MIN
(8µs/38µs)
I
DET
S/H
V
4.2V
V
DET
2.5V
2R
I
DET
DET
0.3V
0.3V
R
PWM
Current Limit
DET OVP
Latched
2ms
30µs
Valley
Detector
HV
8
I
HV
Timer
55ms
Starter
1st
Valley
27V
FB OLP
t
OFF-MIN
+9µs
Internal
OTP
OVP
t
OFF-MIN
for H version
Latched
+5µs
Latched
VDD
6
Two Steps
UVLO
16V/10V/8V
Latched
Internal
Bias
SET
S
CLR
R
DRV
Q
Q
18V
5
GATE
4
GND
7
NC
Figure 2. Functional Block Diagram
© 2009 Fairchild Semiconductor Corporation www.fairchildsemi.com
Rev. 1.0.2 • 5/21/10 2
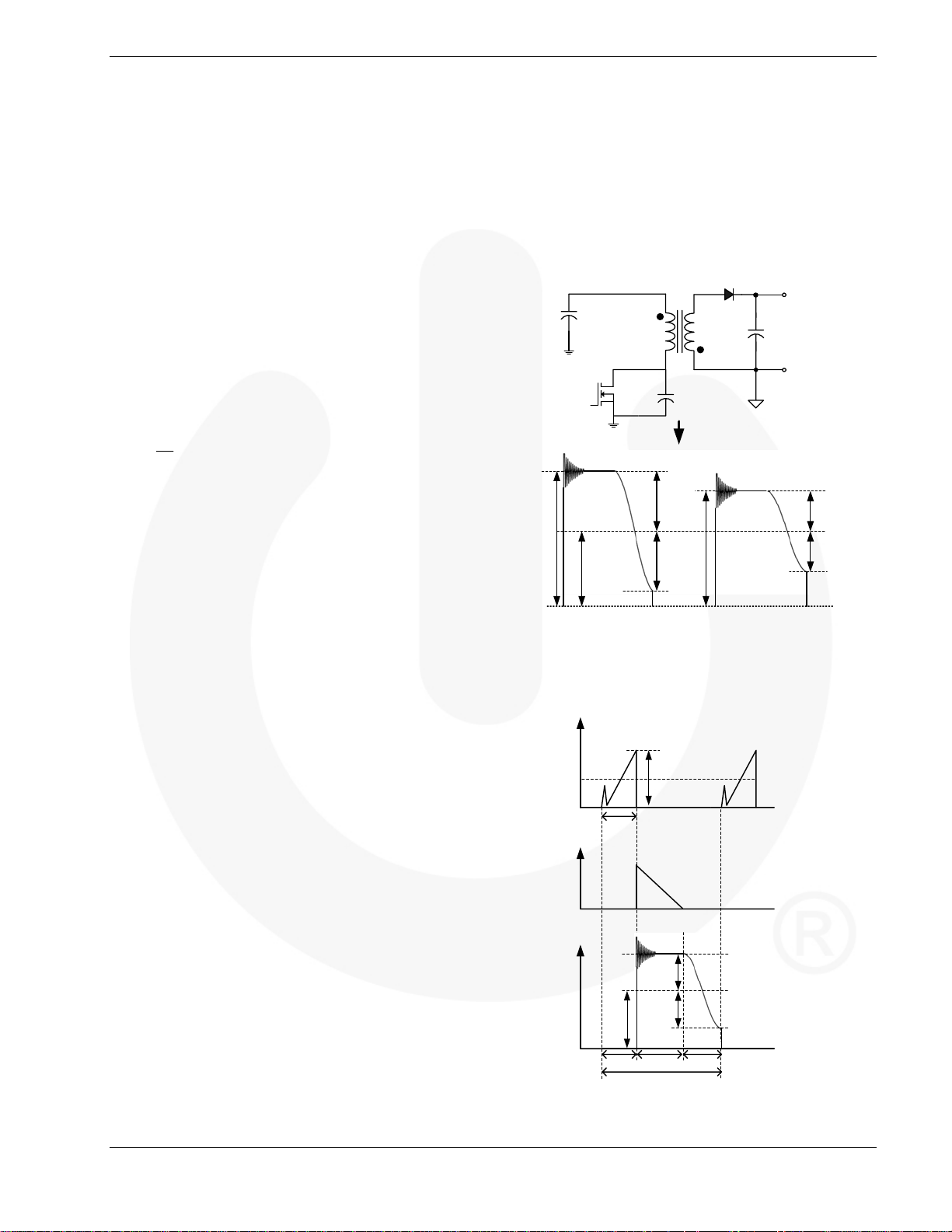
AN-6300 APPLICATION NOTE
Design Procedure for the Primary-Side Inductance of Transformer
In this section, a design procedure is described using the
schematic of Figure 1 as a reference.
[a] Define the System Specifications
Line voltage range (V
Maximum output power (P
Output voltage (V
o
in,min
and V
).
o
in,max
)
) and maximum output current (Io)
Estimated efficiency (η)
The power conversion efficiency must be estimated to
calculate the maximum input power. In the case of NB
adaptor applications, the typical efficiency is 85%~90%.
With the estimated efficiency, the maximum input power
is given by:
P
P=
in
o
η
(1)
designed to turn on the MOSFET when V
minimum voltage V
-n(Vo+Vd).
in
n:1
+
V
in
-
n(Vo+Vd)
C
oss
-
+
+-
+
V
ds
-
V
d
reaches its
ds
+
V
o
-
[b] Estimate Reflected Output Voltage
Figure 3 shows the typical waveforms of the drain voltage
of quasi-resonant flyback converter. When the MOSFET
is turned off, the DC link voltage (V
output voltage (V
Schottky diode (V
) and the forward voltage drop of the
o
) reflected to the primary, are imposed
d
on the MOSFET. The maximum nominal voltage across
the MOSFET (V
VV+nV+V=()
ds,max in,max o d
ds
) is:
where the turns ratio of primary to secondary side of
transformer is defined as n and V
), together with the
o
is as specified in
ds
(2)
V
ds
V
in,max
0V
n(Vo+Vd)
n(Vo+Vd)
n(V
)
n(V
o+Vd
o+Vd
)
V
ds
Figure 3. Typical Waveform of MOSFET Drain Voltage
for QR Operation
I
ds
Equation 2.
pk
I
By increasing n, the capacitive switching loss and
I
in
ds
conduction loss of the MOSFET is reduced. However, this
increases the voltage stress on the MOSFET as shown in
Figure 3. Therefore, determine n by a trade-off between
I
DT
d
s
the voltage margin of the MOSFET and the efficiency.
Typically, a turn-off voltage spike of V
100V, thus V
is designed around 490~550V
ds,max
is considered as
ds
(75~85% of MOSFET rated voltage).
V
[c] Determine the Transformer Primary-side
Inductance (L
)
P
ds
Vin+n(Vo+Vd)
n(Vo+Vd)
Figure 4 shows the typical waveforms of MOSFET drain
current (I
MOSFET drain voltage (V
t
, the current flows through the secondary side rectifier
OFF
diode. When I
resonance between the effective output capacitor of the
MOSFET and the primary-side inductance (L
minimize the switching loss, the FAN6300/A/H is
), secondary diode current (Id), and the
ds
) of a QR converter. During
ds
reduces to zero, Vds begins to drop by the
d
). To
P
n(Vo+Vd)
V
in
t
t
ON
OFF
T
S
t
F
Vin-n(Vo+Vd)
Figure 4. Typical Waveform of QR Operation
© 2009 Fairchild Semiconductor Corporation www.fairchildsemi.com
Rev. 1.0.2 • 5/21/10 3
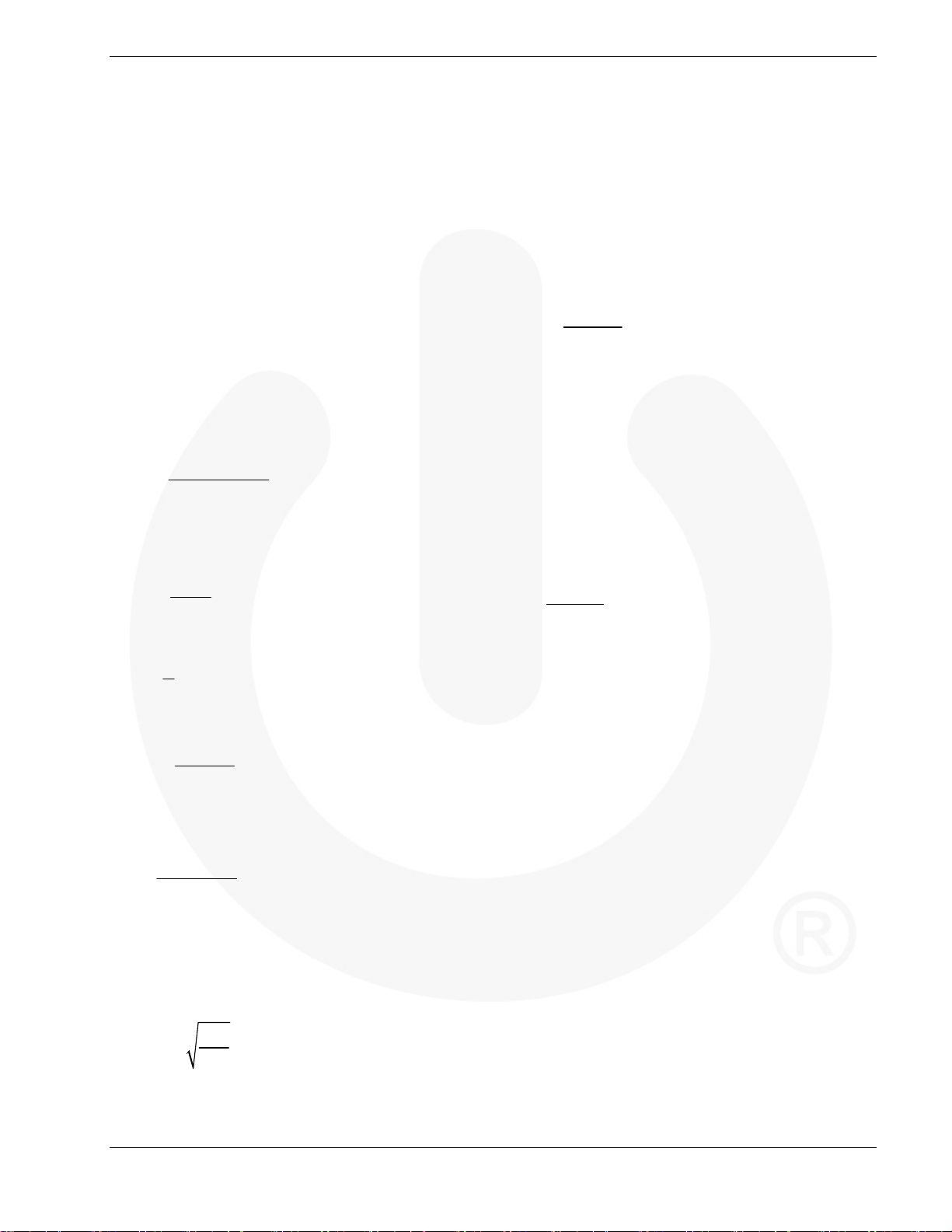
AN-6300 APPLICATION NOTE
To determine the primary-side inductance (LP), the
following variables should be determined beforehand:
The minimum switching frequency (f
s,min
): The
maximum average input current occurs at the
minimum input voltage and full-load condition.
Meanwhile, the switching frequency is at minimum
value during QR operation.
The falling time of the MOSFET drain voltage (t
):
f
As shown in Figure 4, the falling time of MOSFET
drain voltage is half of the resonant period of the
MOSFET effective output capacitance and primaryside inductance. If a resonant capacitor is added to be
paralleled with C
, tf can be increased and EMI can
oss
be reduced. However, this forces a switching loss
increase.
application is about 0.5~1μs.
After determining f
The typical value of t
and t
s,min
, the maximum duty cycle is
f
for NB adaptor
f
calculated as:
nV+V
()
D(1-ft)
=
max s,min f
where V
od
n V +V +V
()
od in
is specified at low-line and full-load.
in,min
××
(3)
According to Equation 1, the maximum average input
current I
I
in,max
According to Figure 3, I
IDI
in,max max d s,max
I
ds,max
I
ds,max
In Equation 5, replace I
combine Equations 4 and 5 to obtain L
L
=
P
where P
respectively, and f
is determined as
in,max
VI
oo
=
V η
in,min
in,max
1
=
k
(5)
2
pk
can be determined as:
VD
pk
in,min max
=
Lf
(V D
in,min max
2P f
in s, min
, and D
in
ms,min
2
)
are specified in Equations 1 and 3,
max
s,min
(4)
can be obtained as:
(6)
pk
by Equation 6, then
ds,max
P:
(7)
is the minimum switching
frequency.
Once L
is determined, the RMS current of the MOSFET
P
in normal operation are obtained as:
D
rms peak
II
ds,max ds,max
max
=
3
(8)
[d] Determine the Proper Core and the
Minimum Primary Turns
When designing the transformer, consider the maximum
flux density swing in normal operation (B
). The
max
maximum flux density swing in normal operation is
related to the hysteresis loss in the core, while the
maximum flux density in transient is related to the core
saturation.
From Faraday’s law, the minimum number of turns for the
transformer primary side is given by:
pk
LI
N10
P,min
Pds,max
=
BA
max e
6
×
(9)
where:
L
is specified in Equation 7;
P
pk
I
is the peak drain current specified in Equation 6;
ds,max
A
is the cross-sectional area of the core in mm2; and
e
B
is the maximum flux density swing in tesla.
max
Generally, it is possible to use B
=0.25~0.30 T.
max
Determine the Number of Turns for Auxiliary
Winding
The number of turns for auxiliary winding (Na) can be
obtained by:
V+V
DD D1
N=
a
V+V
od
(10)
where:
V
is the operating voltage for VDD pin;
DD
V
is the forward voltage drop of D1 in Figure 5; and
D1
V
and Vd as determined in Equation 2.
o
© 2009 Fairchild Semiconductor Corporation www.fairchildsemi.com
Rev. 1.0.2 • 5/21/10 4