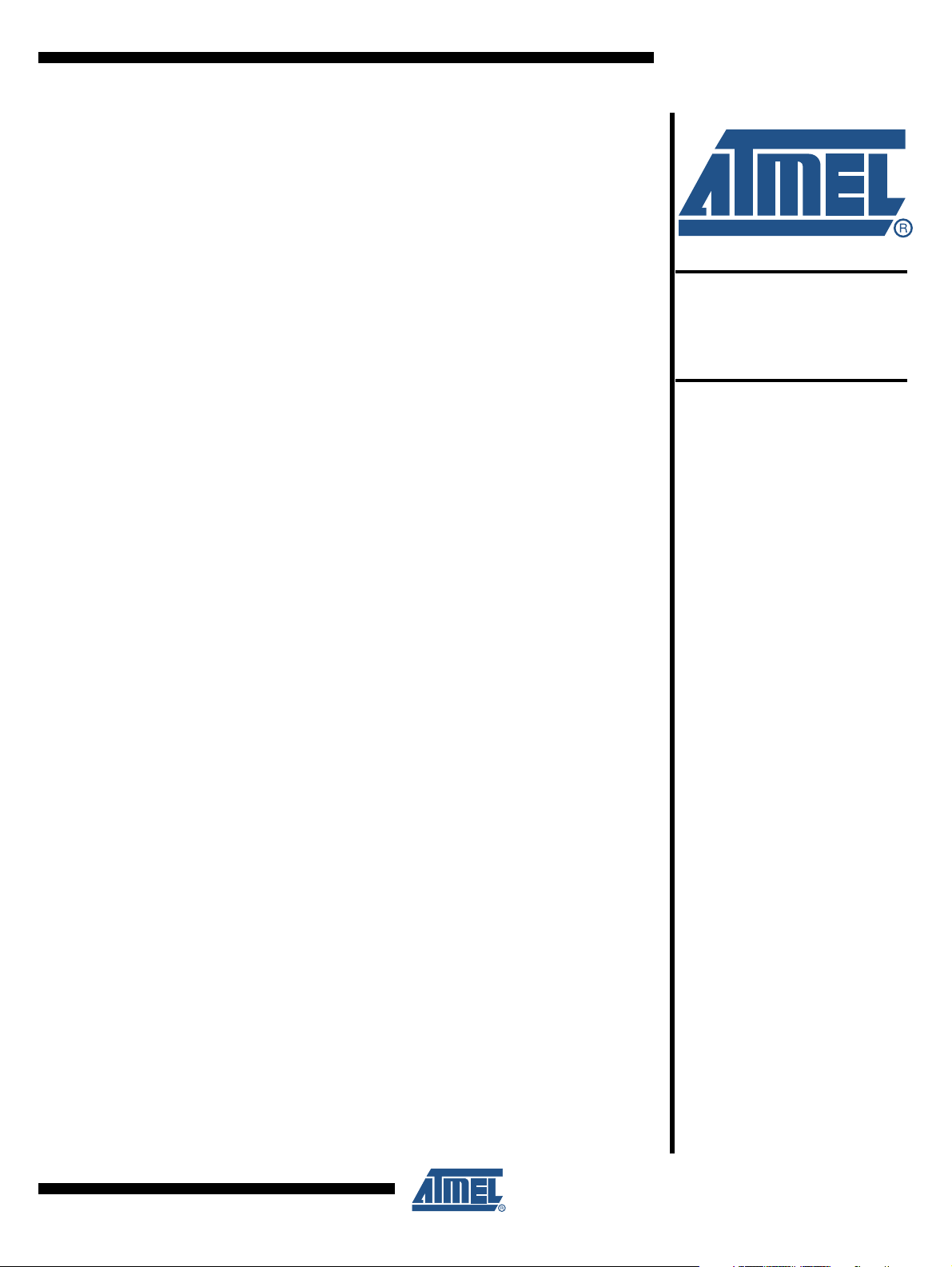
Effect of RF System Parameters on Receiver
(ATA5745/ATA5746) Sensitivity
1. Introduction
In short-range RF system design, focus is typically placed on transmitter output power
and receiver sensitivity to establish a high-quality RF link. However, due to regulatory
requirements limiting transmitter output power and the plethora of devices that easily
achieve them, often it is the receiver’s sensitivity which remains as the key system
parameter of interest when designing an RF wireless application.
ATA5745
ATA5746
Application Note
Given the choice between two receivers priced the same, what’s the better choice, a
device with a sensitivity of –110 dBm (OOK at 2 Kb/s with a BER of 10
sensitivity of –112 dBm (ASK at 9.6 Kb/s with a BER of 10
to select the device with the best sensitivity number. But, without a theoretical and
empirical understanding of the assumptions used to measure sensitivity, a meaningful
side-by-side comparison of the devices on sensitivity alone becomes nearly
impossible.
A thorough understanding of what receiver sensitivity means, how it is measured, and
how it is affected by other RF system parameters, provides the engineer with a solid
foundation upon which to make design decisions that will result in RF system performance that meets or exceeds the design objection.
The purpose of this document is to explain the concept of RF receiver sensitivity as
well as associated terms such as BER and jitter. These terms will be combined with
other common RF system parameters such as data rate, carrier frequency and modulation format to explore how they interact with and affect the RF receiver performance
of Atmel
®
’s highly integrated UHF ASK/FSK receiver ATA5745.
–3
)? One might be inclined
–2
) or with a
9174A–AUTO–01/10

2. Super Heterodyne Receiver
LNA LPF DATA
F
RF
F
LO
FRF - F
LO
IF AMP
Local
Oscillator
Detector
Demodulator
The most common receiver configuration found today in short-range RF wireless applications
is the super heterodyne architecture. The basic principle of operation is the translation of
received RF signals to an intermediate frequency band where the weak input signal is amplified before being applied to a detector. This is achieved by mixing a local oscillator signal FLO
with the received signal FRF to produce an output consisting of FRF + FLO and FRF – FLO. A
lowpass filter typically rejects FRF + FLO and leaves FRF – FLO for further amplification and
filtering. The result is a replica of the modulated RF spectrum that appears translated to a
lower frequency domain called the intermediate frequency (IF). Finally, the detector/demodulator strips off the IF signal and converts what's left into a digital data stream for processing. A
block diagram depicting this general principle is shown in Figure 2-1.
Figure 2-1. Super Heterodyne Receiver Block Diagram
3. Receiver Sensitivity Terminology
In simple terms, receiver sensitivity is defined as the minimum amount of signal power
required at the input of a receiver that results in an accurately demodulated signal “MOST of
the time”. The criterion that defines “MOST of the time” is bit error rate. Input signal power is
expressed in dBm while bit errors are expressed as a rate, usually 10
1000 bits.
There is very little in the way of ambiguity when it comes to measuring signal power applied to
the input of a receiver. RF signal generators are capable of providing accurate output powers
into a standard 50Ω load.
However, when it comes to defining a bit error, there is much more to consider. One can
define a bit error in several different ways. One simple way to measure a bit error rate would
be to count the total actual number of demodulated pulses over a given interval of time and
compare them by the total theoretical number of demodulated pulses over the same interval.
This approach isn’t acceptable because it does not detect changes in pulse width, a phenomenon that may occur as the RF signal grows weaker. For example, Figure 3-1a shows a
theoretical 8-bit modulation stream. Figure 3-1b shows a possible demodulated bit stream at
the output of a receiver. Clearly, the output of the receiver shown in Figure 3-1b would not be
considered acceptable, but according to the bit error criteria defined above, it would not have
any bit errors because both Figure 3-1a and Figure 3-1b possess the same number of pulses
during the defined interval.
-3
or 1 error per every
2
ATA5745/ATA5746
9174A–AUTO–01/10

Figure 3-1. Bit Pattern
a
- Reference bit pattern
b - Pulse widt variation jitter, +
Δ
t
1
+Δ t
1
-Δ t
2
c - Phase variation jitter, -Δ t
2
123 45678
123 45678
123 45678
ATA5745/ATA5746
123 45
123 45678
To reconcile this disparity, the concept of “jitter” must be introduced and applied to the bit error
criteria. For the case of demodulated data from an RF receiver, jitter is the unwanted variation
of phase or pulse width in the demodulated signal. Some examples of jitter are shown in Fig-
ure 3-2. Figure 3-2a shows the reference bit pattern and Figure 3-2b and Figure 3-2c show
pulse width and phase jitter, respectively.
Figure 3-2. Examples of Jitter
678
a - Theoretical bit pattern
b - Possible output bit pattern
A common approach for defining a bit error is to create a time limit, ±Δt, that is a percentage of
the edge to edge bit period, T. Using this approach, a bit error would be any bit edge transition
that occurs outside of the time limit window ±Δt. An example of the bit error rate jitter window is
shown in Figure 3-3. In this case, jitter of ±25% is depicted. For the balance of this document,
the default assumption for BER will be 10
-3
with a ±25% jitter window.
Figure 3-3. Reference Signal with Jitter Windows Defining a Bit Error
T
+Δ t
-Δ t
1
Jitter window Jitter window
9174A–AUTO–01/10
T
1
-Δ t2+Δ t
2
3

4. Sensitivity Measurements
Several instruments are needed to perform sensitivity measurements; an RF signal generator,
a modulation source, a bit comparator, and a frequency counter. All are common instruments
in a modestly equipped RF lab, with the possible exception of the bit comparator. Please refer
to Figure 4-1 for a configuration drawing showing the interconnection of these instruments.
Figure 4-1. Sensitivity Measurement Set-up
Modulation
Source
F
MOD
Bit
Comparator
F
MOD
F
F
DEMOD
ERROR
Frequency
Counter
F
MOD
RF Signal
Generator
F
RF
RF
IN
DATA
Receiver
Under Test
OUT
The bit comparator used to gather sensitivity data contained in this document is a custom
design and is not commercially available. In simple terms, it generates a pulse, F
ever the demodulated signal, F
respect to the reference signal, F
, exceeds the jitter window (set to ±25% as default) with
DEMOD
. See previous section titled Receiver Sensitivity Termi-
MOD
ERROR
, when-
nology for more details and definitions of jitter and jitter window. The Bit Error Rate is
calculated by dividing the bit comparator’s error signal, F
, by the reference signal, F
ERROR
MOD
Additionally, the bit comparator has provisions to enable the adjustment of the jitter window
from ±10% to ±55%.
The modulation source consisted of a square wave signal whose duty cycle was maintained at
50% and whose frequency was varied. A commonly accepted data bit encoding standard for
low-cost and low data rate RF systems that conforms to this signal definition is Manchester. In
many applications, Manchester yields optimum receiver performance by virtue of the characteristic average DC level of 50% that is present on the demodulated signal.
A pictorial representation of Manchester data bit encoding is supplied in Figure 4-2a and Fig-
ure 4-2b. The bit frame period, T
BIT FRAME
bit “0” appears as a falling edge during T
during T
BIT FRAME
. A key observation to note is that the data bit pattern affects the frequency of
, is defined as the reciprocal of the data rate. A data
BIT FRAME
while a data bit “1” appears as a rising edge
the modulation signal. When alternating data bit polarities are used, e.g., “0101”, the resultant
modulation signal frequency is half the data rate. However, when consecutive “1”s or consecutive “0”s used, the modulation signal frequency is equal to the data rate. In this document, it
was assumed that consecutive data bits of the same polarity were used to generate the modulation signal. This means that a data rate of 1 kB/s will require a modulation source of 1 kHz.
.
4
ATA5745/ATA5746
9174A–AUTO–01/10
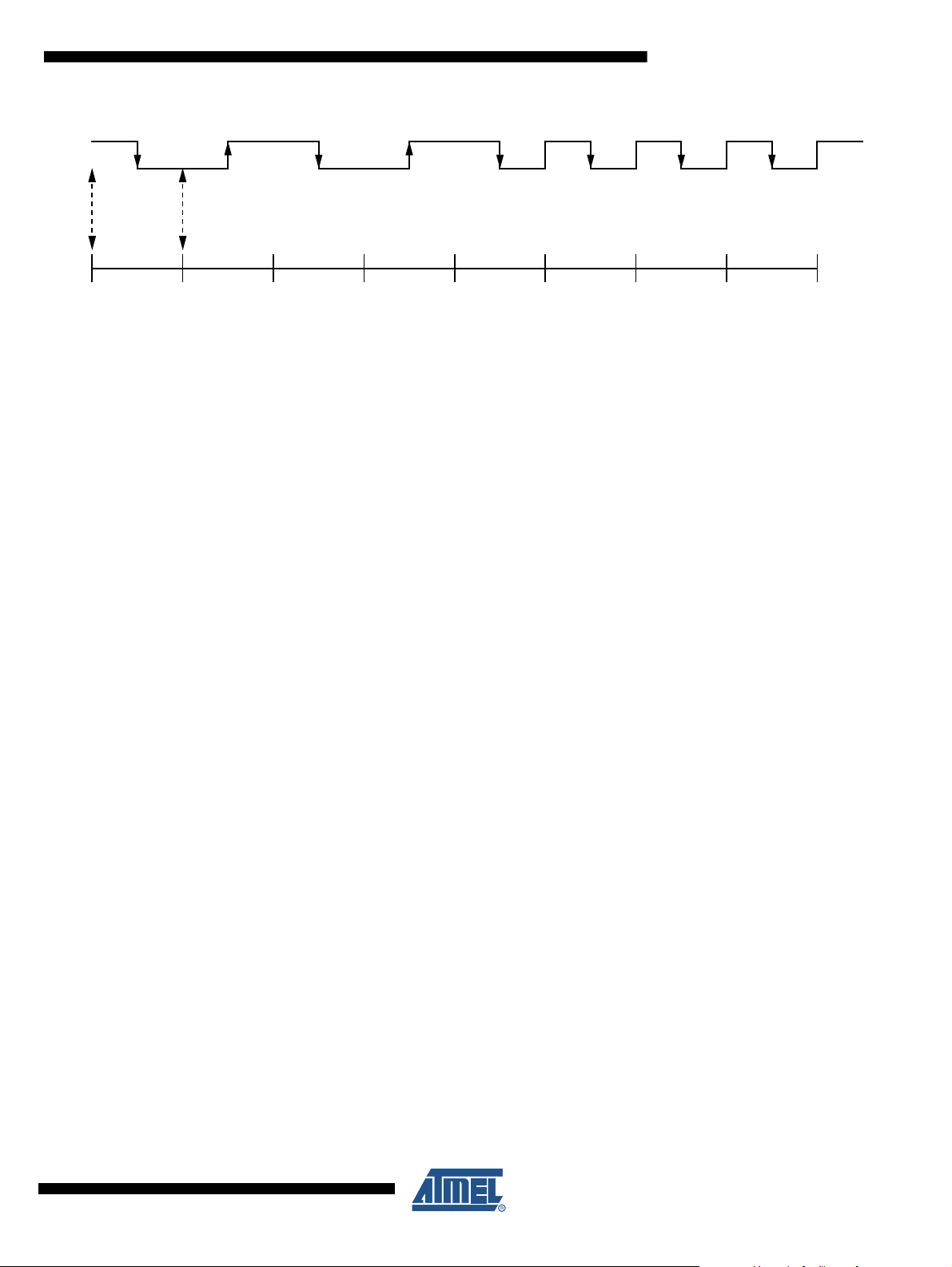
Figure 4-2. Pictorial Representation of Manchester Data Bit Encoding
“0”“1”
T
BIT FRAME
“0”“1”“0”“0”“0”“0”
a - Modulation Signal
b - Data Bit Pattern and Bit Frames
The ATA5746 (315 MHz) reference design was used in this document to gather the typical
data and to show relationships between measured sensitivity as a function of various RF system parameters. Sensitivity data for each graph was obtained from a sample population of ten
boards. The data points shown are based on an average of this population. The trend lines
interpolate behavior between data points and utilize a TBD (e.g., linear, 1st order, log, etc.) to
create best fit. Caution should be exercised before assuming that the results observed on the
Atmel receiver can be extended to receivers from other suppliers without first verifying the
results.
ATA5745/ATA5746
9174A–AUTO–01/10
5