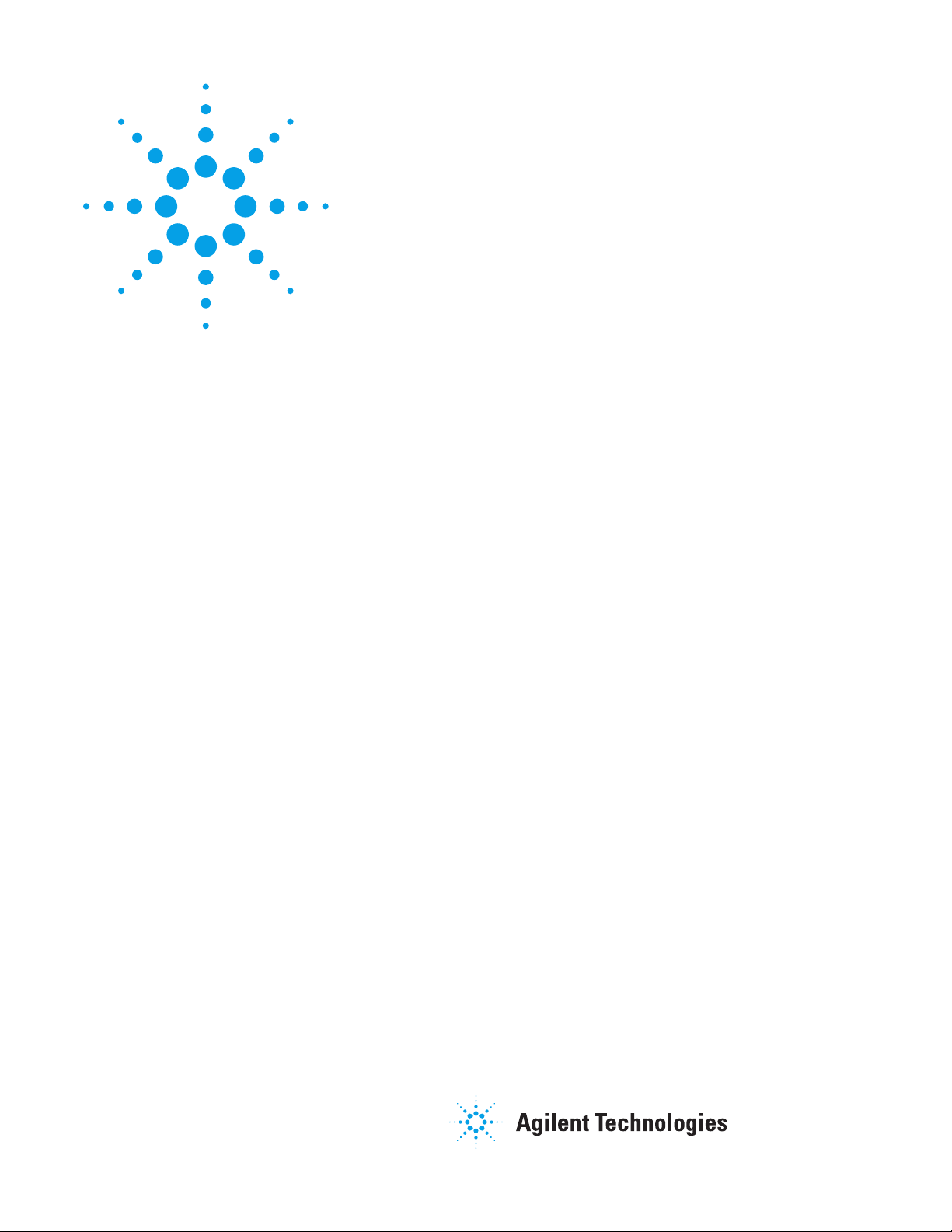
The Agilent Ion Mobility Q-TOF Mass
Spectrometer System
Technical Overview
The Agilent Ion Mobility Q-TOF Mass Spectrometer
System
• Delivers an added dimension of separation
• Provides direct measurement of accurate collision cross sections
• Preserves structural characteristics of molecular conformations
• Expands coverage maps for complex samples.
Introduction
The Agilent 6560 Ion Mobility Quadrupole Time-of-Flight (IM-QTOF) LC/MS system
enables high performance ion mobility and very precise and accurate collision cross
section (CCS or W) measurements without class dependent calibration standards. The
Agilent mobility device operates under uniform low field conditions, thus allowing the
drift time information for ions to be directly converted to collision cross section information. The innovative ion funnel technology in this instrument dramatically increases
the ion sampling into the mass spectrometer and results in higher quality MS/MS
spectra at trace levels.
The Agilent IM-QTOF system is the first commercially available uniform field ion
mobility system, which coupled with the Agilent 1290 UHPLC, provides the combined
separation power and selectivity of LC, IM, and MS techniques. Laboratories involved
in cutting edge research can speed up research programs and have greater confidence in compound identification with the additional dimension of separation as well
as the structural information provided by ion mobility measurements. This instrument
is the only commercially available drift tube ion mobility high resolution (both mobility
and mass) LC/MS system that simultaneously provides high sensitivity and accurate
collision cross section measurements.
Authors
Ruwan Kurulugama, Ken Imatani, and
Lester Taylor
Agilent Technologies, Inc.
Santa Clara CA
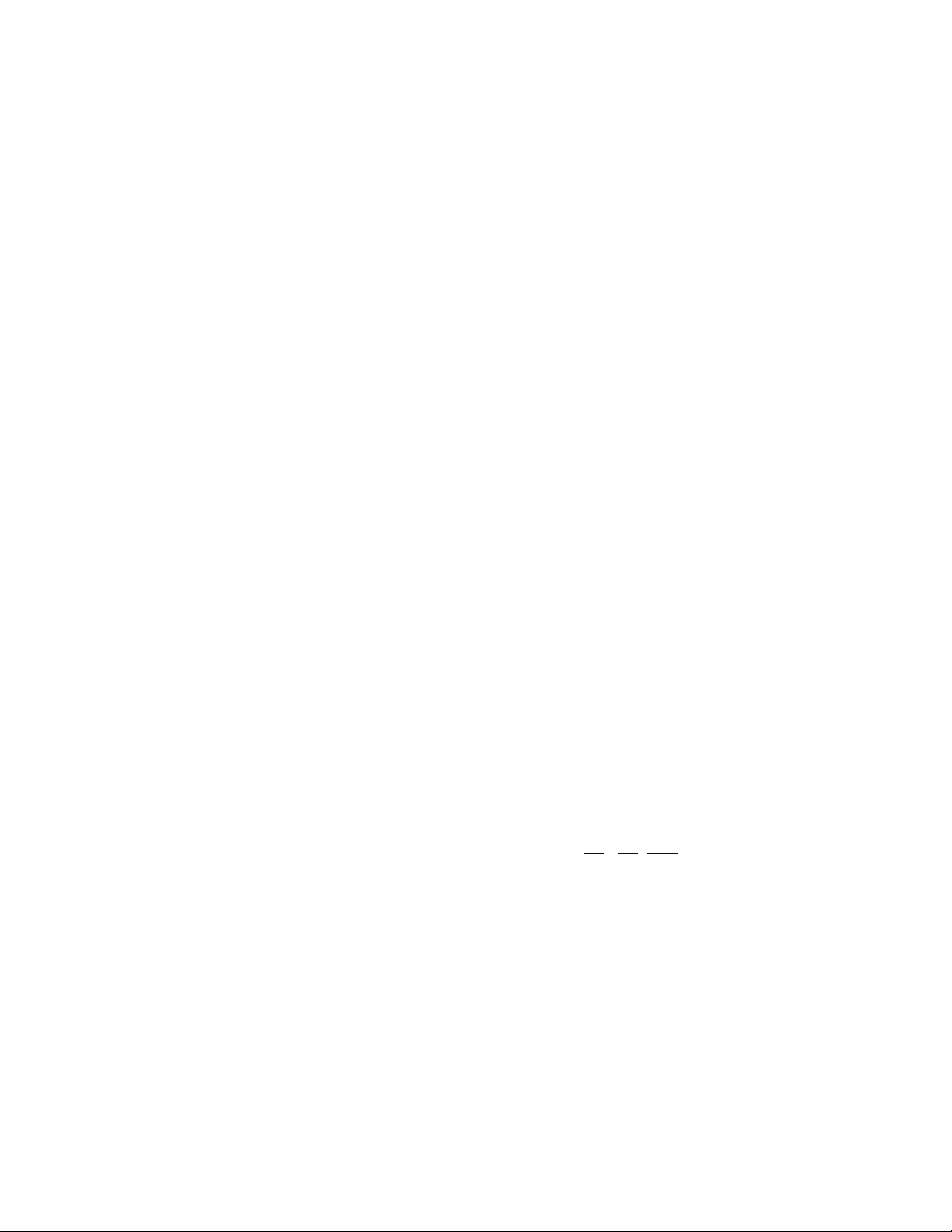
The Agilent ion mobility system was developed with the collaboration of scientists
from a number of academic institutions and government laboratories. In multiple studies, the instrument has demonstrated the ability to reveal significantly greater analytical detail for complex samples compared to high resolution mass spectrometry
technology alone.
Researchers have reported that while high resolution mass spectrometry has become
the analytical cornerstone for proteomics, metabolomics, and other research applications requiring the analysis of highly complex samples, there has also been significant
interest in the use of ultra-fast orthogonal techniques to provide added dimensions of
separation. This new ion mobility system will provide researchers with greater
analytical detail than ever before.
Principles of Ion Mobility Separation
In a classical uniform field drift tube, the electric field within the drift cell moves ions
through the device while the drag force due to the collisions of these ions with the
stationary buffer gas molecules acts against the electrical force that moves the ions.
The drag force experienced by the ions depends on their collision cross sections (a
function of size and shape), electrical charge, and mass. Multiply charged ions move
through the buffer gas more effectively than singly charged ones since they experience a greater force due to the electric field. Ions with larger cross sections are
retarded more easily by collisions with the buffer gas in the drift tube. The drag force
resulting from collisions of ions with the buffer gas molecules acts against their
acceleration imposed by the electric field. Thus an equilibrium state is quickly reached
and the ions start moving with constant velocity (Vd) which is proportional to the
applied electric field (E). The proportionality constant (K) is the gas phase mobility of
an ion. The diffusion limited resolving power is dependent upon the length (L) of the
drift cell, electric field (E), charge state of the analyte ions (Q), and the buffer gas
temperature (T).
V
d
= KE
Mobility is a function of the ion’s interaction with the buffer gas, its mass and its electrical charge. Furthermore, the reduced mobility (K
0
) depends on the gas temperature
and the mass of the buffer gas molecules.
2
where L is the length of the drift cell, tdis the corrected drift time, E is the electric field
across the drift cell, P is the pressure of the drift cell, and T is the temperature of the
buffer gas.
273.2
P
L
K0 =
E
t
d
760
T
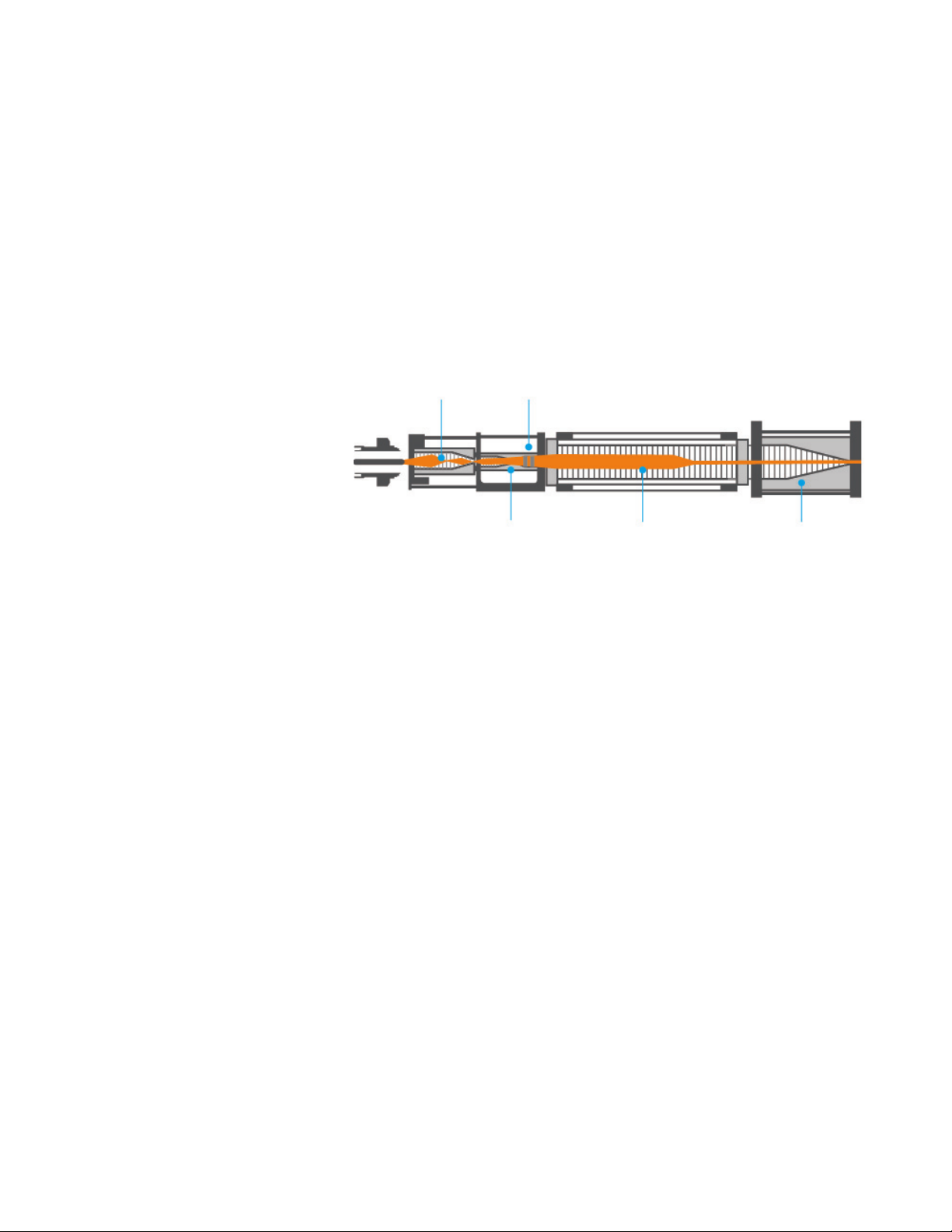
Agilent IM-QTOF System
The Agilent IM-QTOF system provides the following:
• Ion mobility resolving power of greater than 60
• Automated calculation of accurate collision cross sections (~ 1%) without the
need for calibration standards
• High sensitivity for trace level compounds
• Sophisticated data browsing and mining tools
3
Figure 1. Schematic diagram of the ion mobility instrument. Ions generated in the source region are
carried into the front ion funnel through a single bore capillary. The front ion funnel improves
the sensitivity by efficiently transferring gas phase ions into the trapping funnel while pumping away excess gas and neutral molecules. The trapping funnel accumulates and releases
ions into the drift tube. The drift cell is ~80 cm long and generally operated at 20 V/cm drift
field. Ions exiting the drift tube enter the rear ion funnel that efficiently refocuses and
transfers ions to the mass analyzer.
Operation Description
As shown in Figure 1, the Agilent ion mobility system consists of a front funnel, trapping funnel, trapping gate, drift tube, and a rear funnel that couples through a hexapole to the Q-TOF mass analyzer. The front funnel operates at high pressure where
funnel DC and RF voltages propel ions toward the trapping funnel. The key function of
the front ion funnel is to enrich the sample ions and remove excess gas.
The continuous ion beam from the electrospray process has to be converted into a
pulsed ion beam prior to ion mobility separation. The trapping funnel operates by first
storing and then releasing discrete packets of ions into the drift cell.
Ions are separated as they pass through the ion mobility cell based on their size and
charge. Ions with larger collision cross sections undergo higher number of collisions
with drift gas molecules compared to ions with smaller collision cross section.
Therefore, larger ions travel through the drift cell slower than the smaller ions. Also,
ions with higher charge states experience a higher electric force, and hence travel at a
higher velocity, compared to ions with lower charge states. The drift cell is operated
under low field limit conditions allowing the instrument to generate accurate structural information for compounds. Under the low electric field conditions the mobility is
not dependent on the electric field but rather on the structure of the molecule and its
interaction with the buffer gas.
Ions exiting the drift cell are refocused by the rear ion funnel before entering the
hexapole ion guide.
Front funnel Trapping gate
Trapping funnel Drift tube Rear funnel
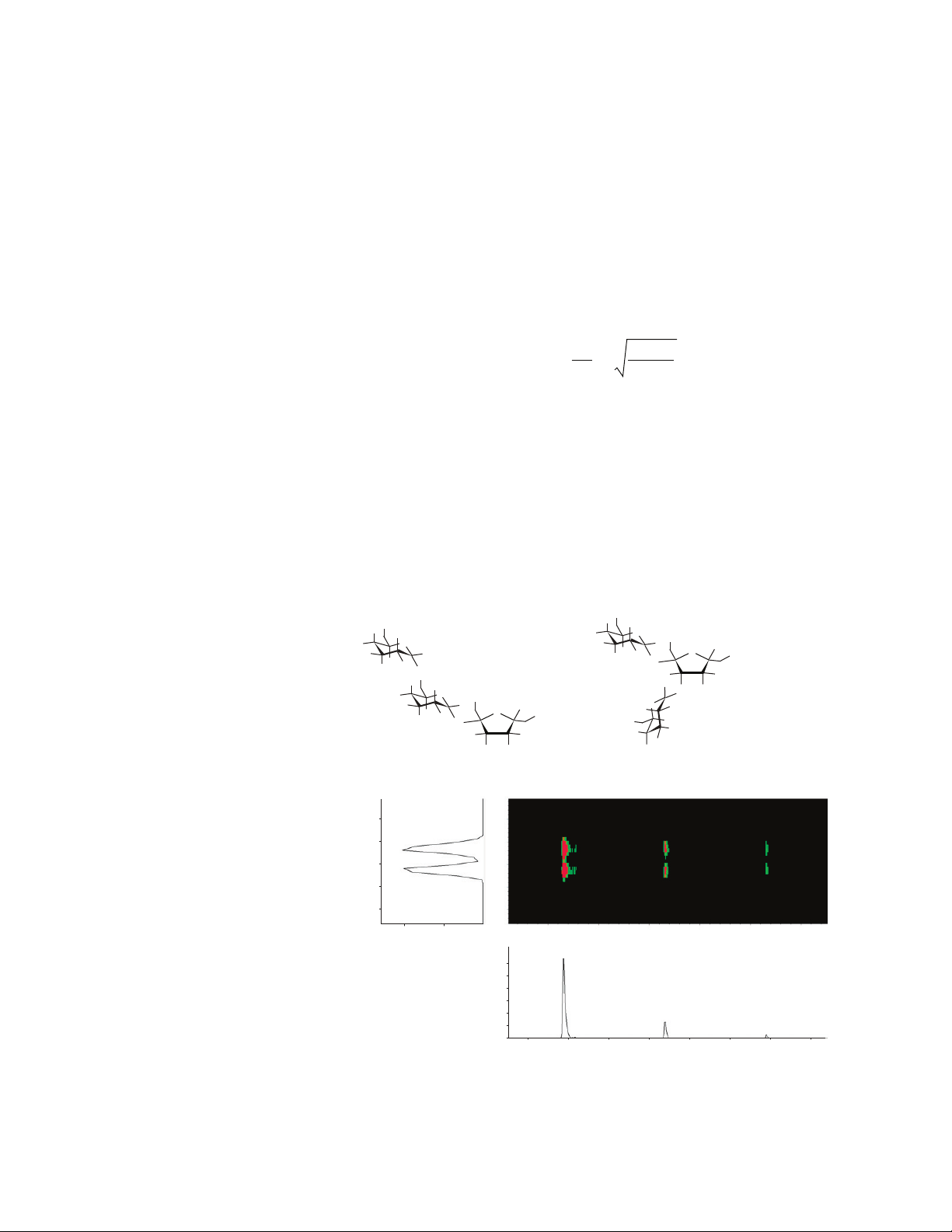
Optimization of Performance - Drift Time Resolution
For ion mobility spectrometry, drift resolution depends on diffusional peak broadening,
width of the initial ion packet, and space charge effects. The most important of these
three factors is diffusional peak broadening. The diffusion limited resolving power is
dependent upon the length (L) of the drift cell, electric field (E), charge state of the
analyte ions and the buffer gas temperature. Longer drift tubes allow ions to drift for a
longer period of time, which results in better ion separation and drift resolution.
4
The Agilent ion mobility drift tube length is approximately 80 cm, and designed to optimize drift resolution and minimize signal loss. Use of a nitrogen buffer gas provides
robust operation at higher drift tube voltages and provides drift resolutions of greater
than 60 for small and large molecules.
Another factor contributing to higher drift resolution is the width of the initial ion
packet. This instrument uses a double grid trapping funnel device to optimize for
higher ion capacity and narrower ion packets. Setting appropriate ion gate pulse times
will determine the number of ions contained in the ion packets which are subsequently injected into the ion mobility cell. The pulse times are typically in the range of
60 to 100 milliseconds.
Figure 2. Separation of isobaric tri-saccharides using the IM-QTOF. A 1:1 mixture of melezitose and
raffinose was infused using a syringe pump. These two carbohydrates can be baseline separated using the ion mobility drift cell and detected using the Q-TOF mass analyzer as sodium
adducts. The ion mobility resolving power for this separation is 60.
t
D
R ==
Dt 16kbTln2
LEQ
OH
H
OH
HO
H
O
H
HO
H
H
OH
H
O
H
H
HO
O
HO
H
H
OH
H
OH
O
HO
H
O
OH
H
H
OH
Raffinose
H
HO
O
OH
HO
H
H
OH
H
O
H
H
O
H
H
HO
H
OH
HO
H
O
OH
H
OH
O
OH
H
Melezitose
×10
1.2
28
27
26
25
24
527.0
5
527.1580
527.5
528.0 528.5 529.0 529.5
28
Raffinose
27
26
25.76 26.68
Drif t time (ms)
25
Melezitose
24
1.0
0.8
0.6
0.4
0.2
0
526.8 527.2 527.6 528.0
528.4 528.8 529.2 529.6
m/z