
INTEGRATED CIRCUITS
74F50728
Synchronizing cascaded dual positive
edge-triggered D-type flip-flop
Positive specification
IC15 Data Handbook
1990 Sep 14
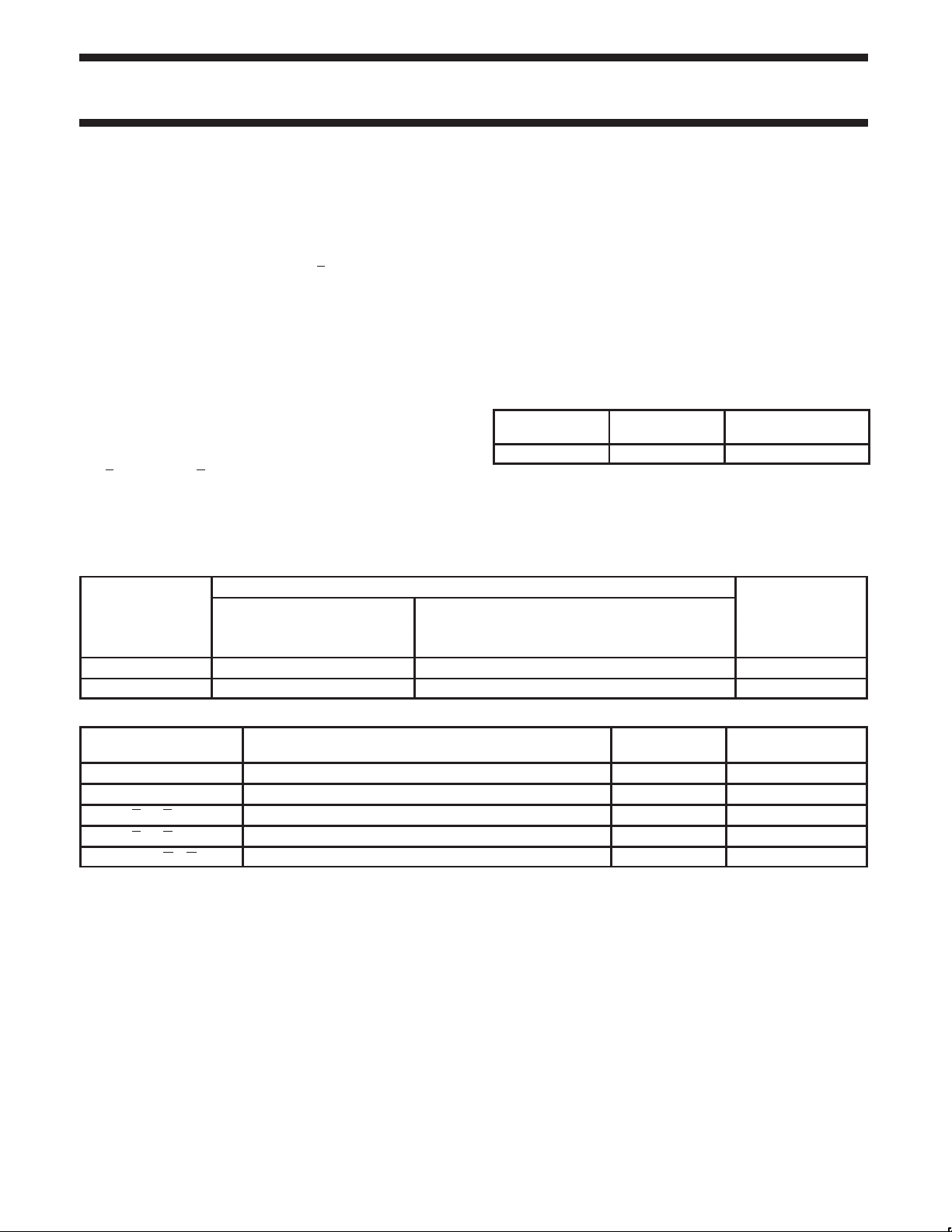
Philips Semiconductors Product specification
Synchronizing cascaded dual positive
edge-triggered D-type flip-flop
FEA TURES
•Metastable immune characteristics
•Output skew less than 1.5ns
•See 74F5074 for synchronizing dual D-type flip-flop
•See 74F50109 for synchronizing dual J–K positive edge-triggered
flip-flop
•See 74F50729 for synchronizing dual dual D-type flip-flop with
edge-triggered set and reset
•Industrial temperature range available (–40°C to +85°C)
DESCRIPTION
The 74F50728 is a cascaded dual positive edge–triggered D–type
featuring individual data, clock, set and reset inputs; also true and
complementary outputs.
Set (S
Dn) and reset (RDn) are asynchronous active low inputs and
operate independently of the clock (CPn) input. They set and reset
both flip–flops of a cascaded pair simultaneously. Data must be
stable just one setup time prior to the low–to–high transition of the
clock for guaranteed propagation delays.
74F50728
Clock triggering occurs at a voltage level and is not directly related
to the transition time of the positive–going pulse. Following the hold
time interval, data at the Dn input may be changed without affecting
the levels of the output. Data entering the 74F50728 requires two
clock cycles to arrive at the outputs.
The 74F50728 is designed so that the outputs can never display a
metastable state due to setup and hold time violations. If setup time
and hold time are violated the propagation delays may be extended
beyond the specifications but the outputs will not glitch or display a
metastable state. Typical metastability parameters for the 74F50728
are: τ ≅ 135ps and T
function of the rate at which a latch in a metastable state resolves
that condition and T
the propensity of a latch to enter a metastable state.
TYPE
74F50728 145 MHz 23mA
≅ 9.8 X 10
0
represents a function of the measurement of
o
TYPICAL f
6
sec where τ represents a
TYPICAL SUPPL Y
max
CURRENT (TOTAL)
ORDERING INFORMATION
ORDER CODE
COMMERCIAL RANGE INDUSTRIAL RANGE
DESCRIPTION
14–pin plastic DIP N74F50728N I74F50728N SOT27-1
14–pin plastic SO N74F50728D I74F50728D SOT108-1
VCC = 5V ±10%, VCC = 5V ±10%,
T
= 0°C to +70°C T
amb
= –40°C to +85°C
amb
PKG DWG #
INPUT AND OUTPUT LOADING AND FAN OUT TABLE
PINS DESCRIPTION
D0, D1 Data inputs 1.0/0.417 20µA/250µA
CP0, CP1 Clock inputs (active rising edge) 1.0/1.0 20µA/20µA
SD0, SD1 Set inputs (active low) 1.0/1.0 20µA/20µA
RD0, RD1 Reset inputs (active low) 1.0/1.0 20µA/20µA
Q0, Q1, Q0, Q1 Data outputs 50/33 1.0mA/20mA
NOTE: One (1.0) FAST unit load is defined as: 20µA in the high state and 0.6mA in the low state.
74F (U.L.) HIGH/
LOW
LOAD VALUE HIGH/
LOW
September 14, 1990 853-1389 00421
2

Philips Semiconductors Product specification
Synchronizing cascaded dual positive
edge-triggered D-type flip-flop
PIN CONFIGURATION
V
SF00605
212
D1D0
14
CC
13
D1
R
D1
12
11
CP1
10
SD1
9
Q1
87
Q1
SF00606
3
6
R
D0
D0
CP0
D0
S
Q0
Q
0
GND
LOGIC SYMBOL
3
4
1
11
10
13
VCC = Pin 14
GND = Pin 7
IEC/IEEE SYMBOL
4
3
2
1
1
2
3
4
5
6
CP0
SD0
RD0
CP1
SD1
RD1
S
C1
1D
R
Q0 Q0 Q1 Q1
56 98
&
74F50728
LOGIC DIAGRAM
4, 10
SDn
Dn
CPn
R
Dn
Vcc = Pin 14
GND = Pin 7
2, 12
3, 11
1, 13
DQ
Q
CP
NOTE: Data entering the flip–flop requires two clock cycles to
arrive at the output.
SYNCHRONIZING SOLUTIONS
Synchronizing incoming signals to a system clock has proven to be
costly, either in terms of time delays or hardware. The reason for this
is that in order to synchronize the signals a flip–flop must be used to
”capture” the incoming signal. While this is perhaps the only way to
synchronize a signal, to this point, there have been problems with
this method. Whenever the flop’s setup or hold times are violated
the flop can enter a metastable state causing the outputs in turn to
glitch, oscillate, enter an intermediate state or change state in some
abnormal fashion. Any of these conditions could be responsible for
causing a system crash. T o minimize this risk, flip–flops are often
cascaded so that the input signal is captured on the first clock pulse
and released on the second clock pulse (see Fig.1). This gives the
first flop about one clock period minus the flop delay and minus the
second flop’s clock–to–Q setup time to resolve any metastable
condition. This method greatly reduces the probability of the outputs
of the synchronizing device displaying an abnormal state but the
trade-off is that one clock cycle is lost to synchronize the incoming
data and two separate flip–flops are required to produce the
cascaded flop circuit. In order to assist the designer of synchronizing
circuits Philips Semiconductors is offering the 74F50728.
DATA
CLOCK
D Q
CP
Q
DQ
CP
DQ
Q
CP
SF00608
Q OUTPUT
Q
Q OUTPUT
5, 9
6, 8
Qn
Q n
10
11
12
13
September 14, 1990
S
C2
2D
R
9
8
SF00607
SF00609
Figure 1.
The 50728 consists of two pair of cascaded D–type flip–flops with
metastable immune features and is pin compatible with the 74F74.
Because the flops are cascaded on a single part the metastability
3

Philips Semiconductors Product specification
Synchronizing cascaded dual positive
edge-triggered D-type flip-flop
characteristics are greatly improved over using two separate flops
that are cascaded. The pin compatibility with the 74F74 allows for
plug–in retrofitting of previously designed systems.
Because the probability of failure of the 74F50728 is so remote, the
metastability characteristics of the part were empirically determined
based on the characteristics of its sister part, the 74F5074. The
table below shows the 74F5074 metastability characteristics.
Having determined the T
and τ of the flop, calculating the mean
0
time between failures (MTBF) for the 74F50728 is simple. It is,
however, somewhat dif ferent than calculating MTBF for a typical part
because data requires two clock pulses to transit from the input to
the output. Also, in this case a failure is considered of the output
beyond the normal propagation delay.
TYPICAL VALUES FOR τ AND T0 AT VARIOUS VCCS AND TEMPERA TURES
T
= 0°C
amb
τ T
VCC = 5.5V 125ps 1.0 X 109 sec 138ps 5.4 X 106 sec 160ps 1.7 X 105 sec
VCC = 5.0V 115ps 1.3 X 1010 sec 135ps 9.8 X 106 sec 167ps 3.9 X 104 sec
VCC = 4.5V 115ps 3.4 X 1013 sec 132ps 5.1 X 108 sec 175ps 7.3 X 104 sec
0
Suppose a designer wants to use the flop for synchronizing
asynchronous data that is arriving at 10MHz (as measured by a
frequency counter), and is using a clock frequency of 50MHz. He
simply plugs his number into the equation below:
MTBF = e
(t’/t)
/TofCf
I
In this formula, fC is the frequency of the clock, fI is the average
input event frequency , and t’ is the period of the clock input (20
nanoseconds). In this situation the f
will be twice the data
I
frequency of 20 MHz because input events consist of both of low
and high data transitions. From Fig. 2 it is clear that the MTBF is
greater than 10
MTBF is 2.23 X 10
T
amb
τ T
= 25°C
41
seconds. Using the above formula the actual
42
seconds or about 7 X 1034 years.
T
amb
0
τ T
74F50728
= 70°C
0
MEAN TIME BETWEEN FAILURES VERSUS DATA FREQUENCY AT VARIOUS CLOCK FREQUENCY
70
10
Clock = 40MHz
Clock = 50MHz
Clock = 650MHz
Clock = 70MHz
Clock = 80MHz
Clock = 100MHz
Data frequency (Hz)
SF00610
Figure 2.
NOTE: V
CC
= 5V, T
60
10
50
Mean time
between failures
(seconds)
1 billion years
= 25°C, τ =135ps, To = 9.8 X 108 sec
amb
10
40
10
30
10
20
10
10
10
00
10
1K 100K 10M
September 14, 1990
4