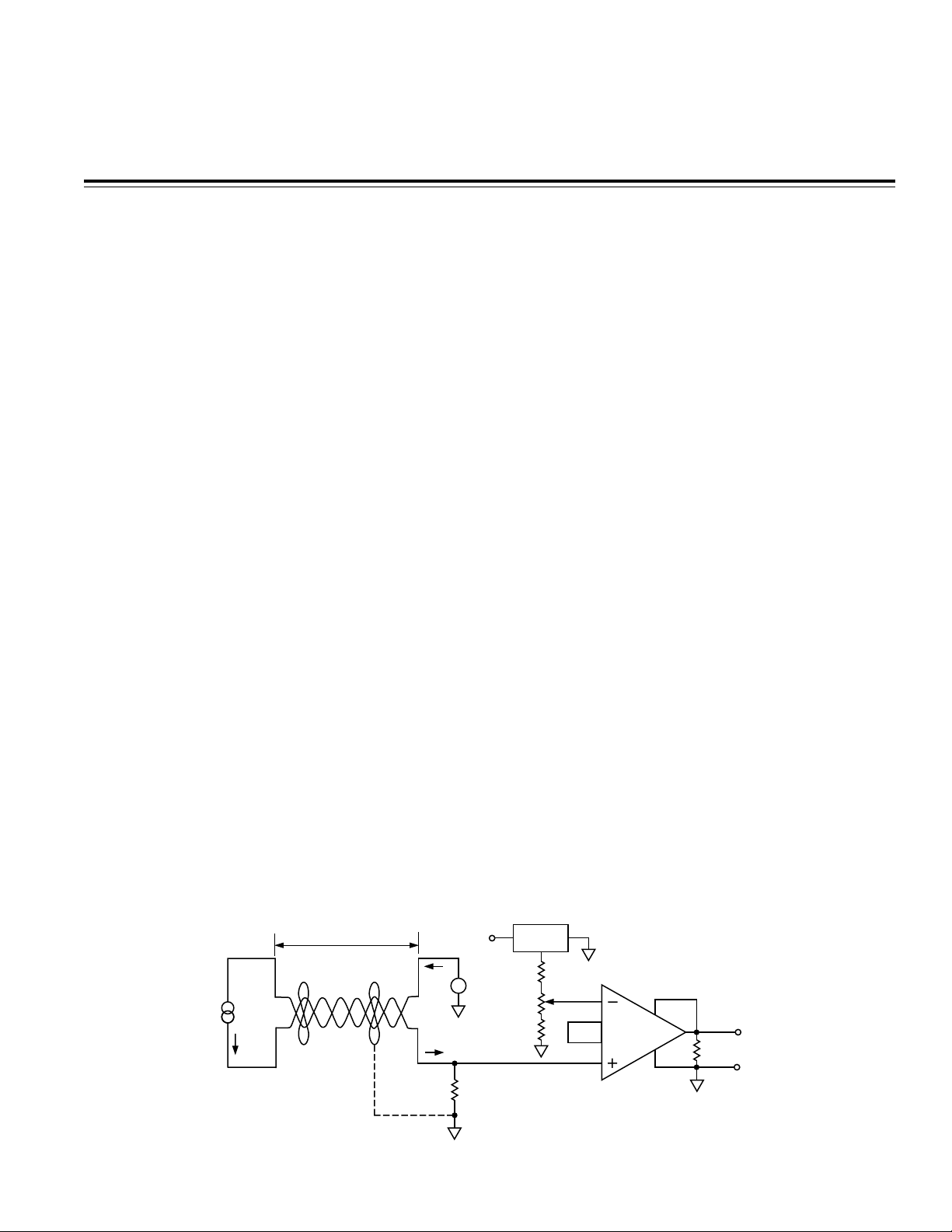
AN-273
a
APPLICATION NOTE
One Technology Way • P.O. Box 9106 • Norwood, MA 02062-9106 • 781/329-4700 • World Wide Web Site: http://www.analog.com
Use of the AD590 Temperature Transducer
in a Remote Sensing Application
by Paul Klonowski
INTRODUCTION
The AD590 is a two-terminal integrated circuit temperature transducer that produces an output proportional to
absolute temperature. For supply voltages between +4 V
and +30 V the device acts as a high impedance, constant
current source supplying 1 µA/K. Laser trimming of the
chip’s thin-film resistors is used to calibrate the device
to an output of 298.2 µA at 298.2K (+25°C).
A typical application for the AD590 is a remote temperature-to-current transducer. Figure 1 shows a thermom-
eter circuit that measures temperature from –55°C to
+100°C and whose output voltage is 100 mV/°C. Since
the AD590 measures absolute temperature (its nominal
output is 1 µA/K), the output must be offset by 273.2 µA
in order to read out in degrees Celsius. The output cur-
rent of the AD590 flows through a 1 kΩ resistance, devel-
oping a voltage of 1 mV/K. The output of the AD580 2.5 V
reference is divided down by resistors to provide a
273.2 mV offset, which is subtracted from the voltage
across the 1 kΩ resistor by an AD524 instrumentation
amplifier. The amplifier provides a gain of 100, so that
the output range corresponding to –55°C to +100°C is
–5.5 V to +10 V (100 mV/°C). An operational amplifier can
substitute for the instrumentation amplifier, although
care must be taken when designing with the
op amp since the gain at the two input terminals will be
different.
THE PROBLEM
A question often asked of Analog Devices Applications
Engineers by customers using the AD590 in a remote
temperature-to-current application is, “How long can I
make the cable and how can I eliminate any noise that
the cable picks up?” Experiments were performed in an
effort to provide some guidelines for answering this
question using the circuit of Figure 1 with a 1000'
shielded, though initially ungrounded, twisted pair cable
(Belden 9461, style 2092). In order to duplicate actual
conditions the experiments were performed in an industrial environment.
TYPES OF NOISE
There are three basic types of noise inherent in a
data-acquisition system. The first type is
noise
: noise received with the original signal and indis-
tinguishable from it. The second type is
transmitted
intrinsic noise:
noise generated within the devices used in a circuit, e.g.,
resistors, op amps, etc. Included in this category are
Johnson, shot and popcorn noise. The third type is
induced noise
: noise picked up from the outside world
and coupled into the circuit. This application note discusses methods of reducing induced noise, which is the
only form of noise that can be influenced by choices of
wiring and shielding.
AD590
I
T
I
T
I
T
+15V
+
7V
–
0.1% LOW
TCR RESISTOR
1mV/K
<
Figure 1. Thermometer Circuit
1kV
AD580
909kV
200V
1kV
G = 100
RG
AD524
2
INSTRUMENTATION AMP
GAIN = 100, 100mV/8C
2kV
E
O
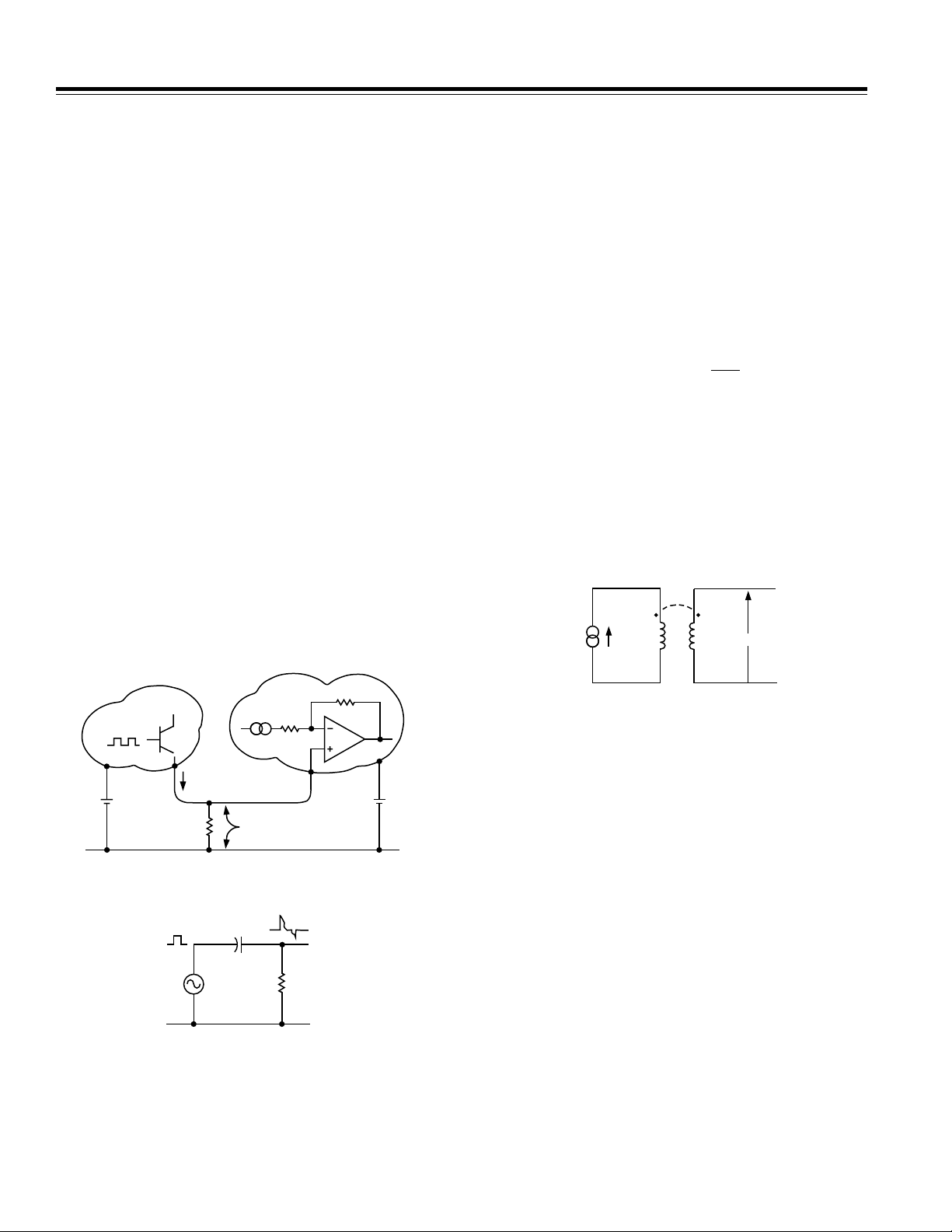
AN-273
NOISE FACTORS
Three elements are involved in any noise problem. The
first is the source of the noise. Possible noise sources
include AM radio signals, logic signals, magnetic fields
and power line transients. The second element is the
coupling medium. That is, how is the noise source entering the circuit? Possible coupling mediums include a
common circuit impedance (Figure 2), stray capacitance
(Figure 3) and mutual inductance (Figure 4). A brief description of each follows.
Common impedance noise is developed by an impedance common to several circuits. This might occur, as
shown in Figure 2, when a pulse output source and an
op amp’s reference terminal are both connected to a
“ground” point having tangible impedance to the
power supply terminal. The noisy return current of Circuit 1 develops a voltage, V
, across the common im-
NOISE
pedance Z which will appear as a noise signal to Circuit
2. Possible solutions to this problem include proper circuits for distributing power and the use of isolation
transformers and optical isolators.
Capacitively-coupled noise is produced by stray capacitance which couples the voltage changing noise source
into high impedance circuits, as shown in Figure 3. The
nature of the impedance Z determines the shape of the
response. Methods of reducing capacitively-coupled
noise include reducing the noise source, properly implementing shields and reducing the stray capacitance.
Magnetically coupled noise is produced by mutual inductance and can occur, for example, in an incorrectly
shielded cable as shown in Figure 7. Figure 4 is a simple,
model of this incorrectly shielded cable, where L
sents the inductance of the shield, L
represents the in-
C
repre-
S
ductance of one of the center conductors, and L
represents the mutual inductance between the two. The
noise current l(t) flows through L
and establishes a
S
magnetic flux; this time-varying flux also surrounds L
and produces a voltage V
rate of change of the current I(t) flowing through L
proportional to the time
NOISE(t)
. This
S
voltage can be expressed as
dI(t)
V
NOISE(t)
=
LM
dt
The third element involved in any noise problem is the
receiver, or the circuit susceptible to the noise. It is important to understand the role of each of the three elements (the noise source, the coupling medium, and the
receiver) in order to solve the noise problem*. In this
experiment it was determined that the noise sources
were 60 Hz pickup and AM radio signals, the coupling
medium was stray capacitance and the receiver was the
AD524.
L
M
L
I
(t)
L
S
V
C
NOISE(t)
M
C
CIRCUIT 2
= I
V
NOISE
NOISE
POWER SUPPLY COMMON
V
S1
CIRCUIT 1
+
I
Z
COMMON
IMPEDANCE
NOISE
Figure 2. Common Impedance Noise
C
S
V
NOISE
SOURCE
Z
Figure 3. Stray Capacitance Noise
Z
V
NOISE
Figure 4. Mutual Inductance Noise
INITIAL NOISE EFFECT
The photograph in Figure 5 shows the output of the circuit in Figure 1 with the shield ungrounded and with the
+
V
S2
remote AD590 at 30°C. Ideally, the output should be a
3 V (100 mV/°C) dc signal. However, a 60 Hz signal result-
ing from an electric field has been capacitively coupled
into the circuit via the stray capacitance of the cable and
then amplified by a gain of 100. Note, however, that the
60 Hz signal is offset by a dc signal; when the output
voltage of the AD524 was measured with a dc voltmeter
the value read was 3.0 V. This is because the voltmeter
read the value of the dc signal due to the AD590 along
with the average value of the 60 Hz sine wave noise signal. The average value of a sine wave is zero. In essence,
notwithstanding the interfering signal the average value
was correct. The accuracy of all the measurements were
verified through the use of an RTD measurement system; the AD590 under test was physically attached to
the RTD.
*An excellent article dealing with this subject is “Understanding Inter-
ference-Type Noise” by Alan Rich, found in Section 20 of the Analog
Devices Databook.
–2–